Carbon Engineering and Occidental Petroleum’s subsidiary 1PointFive, have announced plans to install 70 direct air capture (DAC) plants by 2035 – each capturing up to one million tonnes of carbon dioxide a year. A first plant is expected to be operating by late 2024. If realised, those plants could be capturing just under 0.2% of 2021’s global carbon dioxide emissions. But to prevent catastrophic climate change we’re going to need far more – by one estimate we may need to install as many as 1500 plants each at that 1 million tonne scale, every year between 2030 and 2050.
We also have to capture emissions from plants in hard-to-abate sectors such as steel and cement. The numbers here are perhaps easier to comprehend. The International Energy Agency (IEA) estimates over 1 billion tonnes must be captured every year by 2030 to be in with a chance of meeting net zero by 2050 – requiring a considerable scale up from the 40 million tonnes capacity today. Meeting that target would put a dent in the emissions from 5200 industrial facilities globally that the IEA says are each emitting more than 1 million tonnes of carbon dioxide every year.
How much energy is going to be required, however? The answer is clear: it depends. There are such a broad range of sources of carbon dioxide from diverse industries and types of power plant, that different technologies might be needed for different applications. And in every case a scale-up of supply chains for sorbent materials will be required.
The most widely used mechanism to capture carbon dioxide from industrial flue gases, post combustion, is chemical absorption using amine solvents. Liberating the carbon dioxide from the solvent requires heat, however, and the amount required can make or break the economic feasibility of a process
Monoethanolamine (MEA) is the most commonly used amine solvent for carbon capture. The average reported energy consumption for solvent regeneration is around 1MWh (3.7GJ) per tonne of carbon dioxide captured – enough energy to power the average UK home for 24 days. Innovations are bringing this down to around 722kWh/tonne, according to analysis by energy analytics firm Thundersaid Energy. However, it’s not clear if ‘the solvent cocktails are technically ready for commercial operation’, says founder Rob West.
A power for good
Estimating the energy requirements of producing enough solvent (or solid sorbents) is challenging as details of how much solvent is used and recycled during capture is commercially sensitive. Manufacturing MEA may require around 800kWh/tonne. Norwegian climate foundation Bellona estimates waste from a typical 1 million tonne plant to be 100–300 tonnes per year, implying up to 240MWh could be required to replenish the solvent every year.
The other non-trivial consumer of energy is compression, needed if the carbon dioxide is to be transported or injected underground. ‘In most of the work I’ve seen, the regeneration process is run at close to atmospheric pressure, because the minute you have a pressurised vessel, that becomes much more complicated and expensive,’ says Hannah Chalmers at the University of Edinburgh. ‘You don’t get anything for nothing in thermodynamics.’
Life cycle analyses put the energy required for compression at 111kWh/tonne of carbon dioxide captured, and the energy to inject the gas into a well at 7kWh/tonne.
Taking the IEA’s 2030 target of capturing 1 billion tonnes of industrial carbon dioxide – and assuming the best available solvents – capture, compression and injection energies alone would suggest a minimum of 840TWh would be needed. That’s the equivalent of 5.2% of predicted global renewables generation in 2030, based on current pledges
MOFs’ moment?
The UK’s Drax power station has been developing what it describes as negative emissions technology using woody biomass to replace coal and gas powered units, and has been trialling a range of carbon capture technologies. Its most recent partner is Promethean Particles, which is working with a type of metal–organic framework (MOF). These materials have a large surface area and can be tuned for high specificity to carbon dioxide. When it comes to energy demands, there are no strong chemical bonds to be broken to liberate carbon dioxide, so temperatures of around 80–100°C degrees can be used. This means Promethean can use waste heat to drive the process. ‘It’s really that aspect, which has Drax sufficiently excited to embark on this collaborative journey with us,’ says business development manager, Chris Simmons. Even without the waste heat, early indications are that the energy required to liberate carbon dioxide is around 527kWh/tonne – nearly half that of amine technologies. In theory, using waste heat could take that down by a further 40%, but ‘it’s still early doors’, cautions Simmons.
The volume of sorbent required means scaling up MOF production is essential if carbon capture and storage is going to be rolled out at the magnitude envisaged. Canadian firm Svante has been working with BASF to scale production of its MOF sorbent developed for CCS at cement and hydrogen plants. It says the quantity of sorbent required at a typical cement capture plant is around 200 tonnes. Promethean’s continuous flow technology, means its MOFs can be made more efficiently and at higher throughputs than batch processes. How durable MOF sorbents will be compared with other solid sorbent structures is still unclear, however. The fact that there are no chemical reactions involved in carbon capture using MOFs means the structure will be unaffected, but Promethean is still working to understand the impact of impurities like nitrous and sulfur oxides.
Taking the air
Capturing carbon dioxide from the atmosphere when it makes up just 0.04% of it, is a very different proposition to capturing it from industrial flue gases, where concentrations can range from 4–15%. Consequently, direct air capture (DAC) is in its infancy: 18 facilities operate today, between them capturing just 10,000 tonnes a year.
The largest plant was opened in 2021 by Swiss pioneer Climeworks, and is capturing 4000 tonnes of carbon dioxide a year. The gas is permanently stored by injecting it into water underground where it reacts with the basaltic rock below the plant in Iceland, to form a carbonate mineral. Carbon Engineering’s first plant will be built in the US Permian Basin, in the south of the US, where the carbon dioxide captured will be used to make synthetic fuels and for enhanced oil recovery.
In Carbon Engineering’s chemical looping process, fans pull air onto contactors that have a solution of potassium hydroxide flowing over them. The carbon dioxide in the air reacts with the potassium hydroxide, forming a carbonate salt. Liberating the carbon dioxide involves separating the salt out into pellets, which are heated in a high temperature calcination process – the most energy intensive step of the system. In 2018, David Keith, one of the company’s founders, published a detailed assessment of the energy required for the different steps, which amounted to 2.4MWh/tonne of carbon dioxide with natural gas providing all the heat and electricity requirements. In this assessment the plant also captures an additional 480,000 tonnes of carbon dioxide from the gas power plant providing the energy.
Energy demand fell to 1.8MWh/tonne if the calciner alone were powered by gas and further to 1.5MWh if all processes were entirely powered by electricity. So for those processes alone a 1 million tonne plant powered entirely by electricity would require 1.53TWh/year, or 1.2% of the UK’s renewables generation last year. But Carbon Engineering and 1PointFive intend to create standardised modular designs that can be mass manufactured and allow for swift integration of technology improvements to lower future costs.
Noah McQueen, head of research at carbon capture company Heirloom, has pointed out that the heat and electricity requirements of a DAC plant are similar to those of a petroleum refinery. In the US these facilities use some 880TWh of energy annually. He calculates that allocating that energy to DAC could mean 370 million tonnes of carbon dioxide could be captured each year – or almost 1% of global annual emissions.
Selecting sorbents
Solvent or sorbent recovery rates and lifespan affect energy requirements. Whether scale up of direct air capture is feasible might also depend on those materials’ requirements. Several researchers have carried out life cycle assessments of DAC technologies, but conclusions are uncertain because the data is based on technologies that are not yet at scale, and companies jealously guard the exact details of the materials they’re using.
Sarah Deutz, group leader in energy systems engineering at RWTH Aachen University in Germany, has carried out life cycle assessments using proprietary data from Climeworks, based on the operations of its small-scale modular plants in Switzerland and in Iceland. The analysis was focused on the carbon footprint of plants, using different energy mixes.
Climeworks’ system uses an amine-based solid sorbent. Regeneration of the sorbent and release of carbon dioxide is achieved by applying both low temperature heat and a moderate vacuum. Like Carbon Engineering, its process requires energy to blow air across the contactors.
To assess Climeworks’ process, Deutz chose six promising adsorbents – several of which Climeworks has tested – and calculated the energy required for their production and final disposal, assuming a 95% recycling rate. Energy requirements ranged widely from 39 to 186kWh/tonne of carbon dioxide captured.
Apart from solid sorbents, a DAC plant will require considerable quantities of materials such as steel and cement – that are themselves energy hungry to manufacture. For the present day 4000 tonne plant she calculated that the foundation hall, collectors, process unit and spare parts would add another 44kWh/tonne of carbon dioxide captured to the energy cost of the plant. Recycling all the steel, aluminium and copper could reduce that cost by two-thirds, to 14.4 kWh/tonne of carbon dioxide.
Taking into account all the resource requirements, the plant would use a total of 4MWh/tonne of carbon dioxide, but integrating heat pumps could cut this to 2MWh/tonne.
An assessment based on Climeworks’ optimisation assumptions (including a 60% reduction in sorbent requirement), suggests a facility with a 100,000 tonne/year capacity would require some 1.1MWh/tonne of carbon dioxide captured.
Capturing 1% of global emissions – Climeworks’ target – would mean deploying 3683 such plants. Using the best case (heat pump) scenario suggests they’d require 409TWh of energy. The IEA expects that (based on current pledges), in 2030 electricity generation from renewables will amount to 15,917TWh , so operating DAC at that level would consume 2.5% of expected renewables generation.
Deutz also looked at the materials requirements to achieve such a scale up and concludes that for all materials (except the sorbent), less than 1% of the anticipated output of the market in 2030 would be needed. The picture would be very different if we did have to install 1500 plants 10 times that size each year between 2030 and 2050. 1500 plants would require more renewable energy than is forecast to be generated in 2030.
Driving down costs
Mahdi Fasihi, a researcher at LUT University in Finland who has also modelled the energy costs of DAC systems, notes that there are several opportunities to bring those costs down.
Industrial heat pumps currently in development can provide higher temperatures, while co-siting DAC alongside other industrial processes such as production of synthetic fuels, could allow it to take advantage of waste heat. Similarly, the electrolyser industry is moving to high pressure electrolysis, which also generates heat at temperatures that could be directly used in current low temperature DAC technologies, without the need for heat pumps. At the same time, companies are working to lower the regeneration temperature in the capture process, so eventually a heat pump might not be needed at all.
But a balance must be struck – co-siting DAC plants next to geologic storage, as Climeworks has sought to do, would minimise transportation costs. ‘It’s an optimisation problem,’ says Fasihi. ‘There are certainly many cases where co-locating the DAC plant close to a source of heat or cheap electricity would be beneficial even if it causes other costs, since heat is a significant part of the total cost.’
With an eye on this, Climeworks is partnering with Canadian CCS specialist Svante. Co-locating its DAC plants alongside CCS power plants could allow Climeworks to use waste heat to produce synthetic fuels from the captured carbon dioxide. It’s also working on a US Department of Energy funded project to combine Svante’s MOF sorbent into its modular systems at a renewables generation site in California.
‘In the end,’ says Deutz, ‘direct air capture is not the silver bullet, because we need a lot of energy to do it.’ But the good news is we can still reduce those future requirements by cutting emissions rapidly this decade.
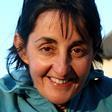
No comments yet