Nina Notman talks to scientists helping to return humans to the moon – for good this time
‘That’s one small step for (a) man, one giant leap for mankind.’ These were Neil Armstrong’s famous words as he became the first person to step on the moon, on 20 July 1969. The US Apollo programme returned astronauts to the moon five more times, with Gene Cernan and Harrison Schmitt becoming the last people to land on the moon in December 1972. No humans have been back for more than 50 years.
For decades, there was little appetite – or funds – for returning crewed missions to the moon. But we are now in a new era of lunar exploration. Nasa’s Artemis programme aims to land humans on the moon in 2025. And many other government space agencies and commercial companies have information-collecting lander missions currently on their way to the moon or scheduled to launch in the next few years.
Nasa’s goal is to build a semi-permanent crewed lunar base, akin to the Antarctic Research Stations. The European Space Agency (ESA), China and Russia have also announced interest in creating lunar research outposts. ‘The moon still has a lot to tell us about the early evolution of the solar system and the solar system’s place in the wider universe,’ explains Ian Crawford, professor of planetary science and astrobiology at Birkbeck, University of London. A permanent human presence on the moon is needed to access the full lunar geological records, he adds.
Moreover, the moon is being used as a testing ground for technology that will eventually take humans to Mars and beyond. ‘The moon is the place to start to learn how to construct scientific research stations off the Earth,’ Crawford says.
Hand luggage only
Keeping astronauts – and their equipment – safe on the moon for extended periods will be an astronomical feat. The longest time humans have spent there so far is three Earth days. The moon is an extremely hostile environment with huge temperature variations, high radiation levels, little atmosphere, constant micrometeorite strikes and huge amounts of abrasive dust.
On top of all that, the high cost of transporting supplies to the moon complicates overcoming these issues. ‘Every kilogram of material you launch from the Earth [to the moon] has a price tag of around a million dollars,’ says Mahesh Anand, a professor of planetary science and exploration at The Open University in Milton Keynes, UK. To make lunar bases viable, the moon’s natural resources will need to be used to reduce the amount of material transported from Earth.
The idea of a near self-sustaining moon base seems preposterous at first glance, since the moon’s surface looks like a barren wasteland. But looks can be deceptive. The first inkling that it might be possible came in 2008 and 2009, when two separate missions found strong evidence for water on the moon. First, India’s Chandrayaan-1 mission found signatures of a very thin layer of water all over the lunar surface. Then water signatures were seen in the plume of debris created when Nasa’s Lunar Crater Observation and Sensing Satellite (LCROSS) slammed into a crater in an area of permanent shadow near the moon’s south pole.
The temperature in the crater hit by LCROSS is around −200°C, and the hope is that it contains water ice. If there, this would be a highly tappable resource. ‘The circumstantial evidence is strong, but absolute proof that a large amount of water is actually in these permanently shadowed craters still needs to be determined,’ says Crawford.
But while frozen water at the poles would provide the easiest access to large amounts of water, the thin layer of water across the entire surface also holds promise. The latest evidence for this water source was found during the analysis of lunar regolith collected by the China National Space Administration’s Chang’e-5 lander in 2020.
Lunar regolith is an abrasive mixture of soil and loose rock on the moon’s surface formed over billions of years by meteorite strikes on the moon’s crust. Anand is part of the Chinese–UK team analysing the regolith collected by Chang’e-5. In March 2023, the scientists reported that they had discovered water trapped in tiny glass beads scattered throughout the regolith. The scientists propose that the water forms when high energy protons in the solar wind strike the beads, reacting with the oxygen present in the glass.
The regolith can be heated – using conventional heating or microwave processes – to release the water from these beads as water vapour. This vapour can then be captured and condensed to collect liquid water.
Breathe easy
Water is not only needed for drinking and growing food, but also for construction and radiation protection; the radiation shielding properties of water have long been known, and some radioactive materials are stored under water on Earth. Water’s biggest use on the moon, however, will likely be as a source of oxygen for breathing and for use as an oxidiser for rocket fuel.
‘You can electrolyse water into hydrogen and oxygen,’ Anand says. Electrolysis is a very well-established method of splitting water into its constituent elements. It has been used for decades both on Earth and aboard the International Space Station, where it is the astronauts’ primary source of oxygen.
Another lunar source of oxygen is the silicates, oxides and other oxygen-containing minerals in the lunar regolith. ‘42–45% of the regolith on the moon is oxygen by weight in different forms,’ explains Jerry Sanders, lead for in situ resource utilisation system capability team at Nasa’s Johnson Space Center in Houston, US.
The main technology being adapted for this purpose is the Fray Farthing Chen (FFC) electrolysis process invented in the late 1990s at the University of Cambridge, UK. This electrochemical process has been commercialised by spin-out company Metalysis, based in Rotherham, UK, into various sized reactors able to generate metal powders from metal oxides. The largest reactor can produce multi-tonnes of powder per year.
The byproducts of the process are carbon dioxide and carbon monoxide. ‘The cathode is the metal oxide, the anode is carbon or graphite and the electrolyte is calcium chloride,’ explains Ian Mellor, Metalysis’s chief scientist and managing director. ‘When we apply a voltage between the two electrodes, the oxygen leaves the cathode, migrates through the electrolyte, combines with the carbon on the anode and is discharged as carbon dioxide or carbon monoxide.’
For use on the moon, Metalysis is developing an inert anode that gives off oxygen gas at this electrode instead. ‘The technology is capable of removing the oxygen from roughly 50 elements in the periodic table in their oxide form,’ says Mellor. Aerospace company Thales Alenia Space’s Harwell site is currently developing a lander that will take a small demonstrator unit to the moon. This lander, being funded by ESA and in partnership with Metalysis, will complete the entire process without human input from the collection of the regolith from the surface through to storing the oxygen until it is needed.
Waste not, want not
There is also an increasing interest in making use of the metal powder byproduct from Metalysis’s oxygen-generating electrolysis technology on the moon. The metal powders can potentially be used for 3D printing construction materials – with 3D printing (additive manufacturing) being one of the uses for Metalysis’s terrestrially-produced metal powders.
Different regions of the moon have different mineral compositions, just like on Earth. A challenge for utilising the metal powers made from lunar regolith will, therefore, be managing the variations in its composition. There are two main forms of lunar regolith which from Earth look like distinct grey and black patches. The grey areas are mostly anorthosites, rich in aluminium, calcium and silicon. The black areas are comprised of basalts including ilmenite, an iron titanium oxide mineral.
Electrolysis isn’t the only tool being developed for accessing the metals in these minerals. Nasa is also looking to make use of several terrestrial processes for stripping metals from these types of materials. Magnetic separation and other techniques suitable for removing iron and titanium from ilmenite are of particular interest, explains Sanders.
Another approach being developed, this time in Anand’s lab, is the use of microwaves to concentrate metals in lunar regolith. His team has found that iron and some other metals can escape their mineral forms when lunar regolith simulants are heated in the microwave. ‘Originally [the metal] is locked up in silicate minerals, but when we microwave them, the iron is moving away from those silicate structures and coming together to become a big blob of metal,’ he explains. These lumps of pure metal could then be extracted using existing techniques, he adds.
A home from home
It isn’t just metal that is needed for lunar construction. Crude regolith also has many potential applications, and government space agencies and commercial companies alike are racing to develop tools for building with it.
‘Just like you see on a construction site on Earth, you have multiple tools and multiple pieces of hardware we can envision that might be equally beneficial on the moon, when you’re trying to build different types of these engineering structures,’ explains Corky Clinton, associate director of the science and technology office at Nasa’s Marshall Space Flight Center in Huntsville, US.
Crude regolith could be used to create habitats with robust protection from radiation and micrometeorites. It will also act as an insulator and stop thermal energy being lost from inside. Landing pads with barriers to protect infrastructure behind them, similar to jet blast deflectors at airports, is another purpose being explored for crude regolith. A lunar base will see resupply rockets coming and going on a regular basis. ‘As a rocket is landing on the moon and the engine plume is firing out a lot of gas, it kicks up a lot of the regolith and this will actually become quite a substantial hazard as these particles will sandblast everything around it,’ says Aidan Cowley, science advisor at the ESA’s European Astronaut Centre in Cologne, Germany.
Making bricks from regolith, and then using robots to build with them, is one approach to building structures on the moon that is being explored. ‘We joke that there could be astronauts building with bricks on the moon, but in reality it is probably going to be an automated robotic system with a lot of human oversight,’ Cowley explains. ‘The astronauts will be acting in a foreman role.’
When you’re on the moon, you want to be as efficient as possible
Longer term, the hope is to eliminate the need for a human supervisor on site. ‘As we proceed into [building] larger and more complex infrastructure, we will definitely want to be able operate continuously for longer periods of time,’ says Clinton. To achieve this, the plan is to move to more autonomous operations that are remotely operated from Earth.
The various techniques being explored to make lunar regolith bricks are all tweaks on terrestrial processes. The simplest approach is to collect regolith, compact it and then sinter it thermally. This can be done in an oven-like structure, by focusing concentrated sunlight, or by using microwave technology or lasers.
Methods to improve brick binding, such as sieving out certain sized materials and the addition of polymers or synthetic binders, are being investigated. Nasa is also developing greener alternatives to Portland cement for the mortar that will hold the regolith bricks together. On Earth, Portland cement production is estimated to generate around 8% of the global carbon dioxide emissions per year. ‘We’re looking at different kinds of cements that we could use on the moon and if successful these could offer a different way of doing things on Earth,’ says Sanders. This idea isn’t without precedent; hundreds of technologies developed for space have gone on to find beneficial uses on Earth, including home insulation, memory foam and baby formula.
Another approach to making regolith bricks is to 3D print them. With additive manufacturing, there is less waste than with traditional methods such as filling moulds. ‘When you’re on the moon, you want to be as efficient as possible,’ says Cowley. The idea is to build up the bricks layer by layer, sintering as they go, perhaps with concentrated sunlight or lasers, to bind the material together, he adds. The crude regolith powder can be printed directly using the laser powder bed fusion technology used to 3D print metal objects on Earth. Alternatively, the crude regolith can be melted and extruded into a filament first, and a fused deposition modelling (FDM) 3D printer used.
Nasa is also working with ICON, a Texas-based construction technology company, to develop super-sized 3D printers able to print entire structures in one go rather than printing individual bricks. ICON is best known for 3D-printing concrete homes, and the company is now adapting its technology to print infrastructure on the moon’s surface using only lunar materials.
Power me up
To supply power to the moon, solar power is the most obvious starting place. The challenge here will be working around the lunar cycle, which lasts 28 Earth days, to provide a constant electricity source over the extended periods of darkness.
This tricky issue is one of the reasons that the south pole has been identified as the target location for the first lunar base, together with the possibility of frozen water and less extreme temperature changes there compared to the Apollo landing site near the equator. ‘There are hilltops [near the lunar poles] that are in near permanent sunlight,’ explains Crawford. ‘They’re within a few kilometres of permanent shadow where the water ice might exist. So, a base located near the south pole would be both close to permanent shadow and its water and close to near permanent sunlight.’ The south pole has been selected over the north pole for scientific reasons. ‘It’s geologically the more interesting,’ Crawford adds.
Nuclear fusion reactors with helium-3 fuel are a second potential energy source for a lunar base. Over billions of years, the solar wind has embedded helium-3 into the moon’s regolith. ‘We know this from the Apollo samples,’ says Crawford, ‘but it’s only present at the parts per billion quantities.’ The feasibility of this idea depends on how much regolith needs to be processed to collect enough helium-3 for a reactor – and whether doing so is economically feasible.
There are other potentially useful resources on the moon, also likely only present in tiny amounts. Along with water signatures, Nasa’s LCROSS mission found evidence of volatiles including methane, ammonia, carbon dioxide, carbon monoxide and hydrogen in the lunar crater it struck. ‘The carbon that might exist there could be very interesting because the rest of the moon is very carbon poor,’ says Sanders. Again, a lot depends on the concentrations, but ‘if you have a carbon source and a hydrogen source, you basically have a petrochemical industry [and] we can start to think about plastic production, binders, polymers, and other things that would support in situ manufacturing and construction’, he adds.
Mission possible
The Artemis programme and the numerous lander missions arriving in the next few years should answer many of the outstanding questions regarding the exact resources present, how they can be extracted, the viability of a lunar base and what it might look like.
Sample return missions will be particularly valuable. Lunar regolith simulants have been used to test all the technology under development, but these materials are currently just educated guesses of the regolith’s actual composition near the south pole. No samples have yet been returned to Earth from this region. ‘There’s a risk there: if we don’t create the simulants to exactly match what we are going to find on the moon, it will affect the processes,’ Clinton explains. Sample return missions will allow the technologies being developed to build with the regolith and tap into its resources to be tweaked to suit the exact composition of the lunar regolith, before the pilot plants start arriving on the moon.
Nasa is aiming to have its first lunar base up and running by around 2030. Whether this happens or not will, according to Crawford, all depend on the success of the SpaceX Starship Lander that Nasa has selected to put humans back on the moon. ‘That vehicle has such an enormous capability in terms of the mass that it can deliver to the lunar surface,’ he says. Its vast cargo hold will allow pilot plants to be landed to start establishing how easy it is to build with, and extract water and metals from, the lunar regolith in situ. ‘The SpaceX Starship Lander will be very enabling if it works as advertised,’ Crawford adds.
Nina Notman is a science writer based in Salisbury, UK
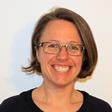
1 Reader's comment