Kit Chapman takes a tour of a US Department of Energy lab, where 3D printing is performed on a massive scale
A Shelby Cobra is parked on the second floor of a building in the US state of Tennessee. It’s painted racing blue and white and its bonnet is signed by Bob Bondurant, a driver who won the Le Mans 24 Hours GT class in a similar model 55 years ago. It’s a fully working classic sports car, though when it was exhibited at a motor show in Detroit, US, in 2015 visitors didn’t pay it much attention. No one realised its parts were 3D-printed.
The Cobra’s home is Oak Ridge National Laboratory’s Manufacturing Demonstration Facility (MDF), one of the most advanced 3D printing labs in the world. ‘We had actually printed cars before,’ says Alexander Plotkowski, a staff scientist at MDF. ‘The problem was that they looked like 3D-printed cars – they have that kind of rough, layered look to them. We did the whole Cobra in about six weeks and finished it so that it’s indistinguishable from a conventional car. We haven’t printed a complete engine, we’re not there yet, but we’re working on it. It’s only a matter of time.’
Grand designs
The car is just one example of how the MDF chemists, metallurgists and engineers are redefining the possibilities of large-scale additive manufacturing, better known as 3D printing. Established in 2012, the lab is a user facility, working in partnership with companies around the world to develop next-generation projects. Many of the main collaborators come from the aerospace industry – such as Boeing, Lockheed Martin and Northrup Grumman – where the rewards from incremental gains are so high they are willing to explore cutting-edge technology.
The MDF’s main workshop, taking up the majority of its one hectare area, resembles a large aircraft hangar with an open plan floorspace occupied by everything from machine tools to CT scanners and electron microscopes – and, of course, 3D printers. These range from small-scale devices on workbenches up to the two-storey Big Area Additive Manufacturing (BAAM) unit – which printed a submersible for the US Navy in 2017. Despite BAAM’s gargantuan size, Oak Ridge has access to something even bigger: the lab collaborates with the University of Maine, US, which in 2019 unveiled the world’s largest 3D printer, capable of printing around 200kg an hour to create objects 30m long, 6m wide and 3m high. Scattered through the hall are polymer chairs – reminders of the time the lab ran out of seats for interns and tasked them with creating their own. Printing time varies depending on the complexity of the part and ranges from minutes to weeks. One of the lab’s longest prints, a single metal component for a helicopter, took three weeks.
Incredible pieces of design can be created, made to specification and meeting challenges that only 3D printing can manage. When the US Office of Naval Research wanted a lightweight underwater arm for its submersibles, standard machine tools couldn’t create in-built channels for the hydraulics. As well as meeting this tricky design brief, the Oak Ridge team printed the arm out of a titanium alloy, making it light enough to toss in the palm of your hand. ‘The inside of this component is hollow,’ Plotkowski says, ‘It’s hollow so that even though this is made out of titanium, it is neutrally buoyant.’
In one corner, the team is working on using additive and subtractive printing to map and fill degraded components such as a crack in a diesel engine or worn turbine blades. As every engine’s topography is unique, Plotkowski explains, crack repair is usually so expensive and complicated the engine is just replaced, creating huge amounts of waste. ‘There’s a multi-billion-dollar industry in remanufactured engines. So being able to do a repair that’s unique every single time is a huge step forward.’ The automotive industry is also using 3D printing to develop parts that could not be fabricated any other way. When tyre manufacturer Michelin wanted to create new all-weather tyres, its engineers designed the ideal tread pattern then used 3D mapping and printing to design a machine tool that could make it.
A few metres away from the titanium robotic arm, dominating the far end of the hall, is a big white box, inside which the lab is designing components for a nuclear reactor; eventually, the idea is that an entire reactor could be printed on demand. Sadly, that’s all the lab can say in the interests of commercial and national security – and some parts of the lab are even more secretive.
At the moment, if you’re going to make a building, you need a printer that’s bigger than the building
The largest section of the room is taken up by a seemingly empty space, occupied only by a few tables with computers with staff standing by. Suspended above their heads are a series of taut wires, a black box hanging from their centre that vaguely resembles the remote control cameras used in sports stadia. It’s the latest project for Brian Post, one of the leads for large-scale thermoplastics, whose team previously designed the world’s first 3D-printed excavator.
The team is now looking at how to print infrastructure sized components out of materials like concrete. Post’s spider-like set up is a mobile, cable-driven 3D printer system that can be whisked anywhere in the world and set up on-site – to print whatever is needed with almost no limit to the size. ‘At the moment, if you’re going to make a building, you need a printer that’s bigger than the building,’ Post explains. ‘The problem is, that’s cost-prohibitive, requiring a giant gantry system and level terrain. [Instead], I can set this up, do all the inverse kinematics and know exactly how to move this around to make different objects, and then I can print what I want. Most construction right now is linear; we make things that are in straight lines because that’s the shape building materials come in. Here we can make complex curvature. We can print architectural features, like complex columns or spiral staircases, at a cost nothing more than it would be to make a straight wall.’
An emerging 3D-printed construction industry already exists, driven by the lower costs for labour and raw materials, and the possibility of creating a home in less than a day. While several different techniques have been pioneered, typically this uses extrusion 3D-printing – essentially squirting the raw material where you wish – with concrete. Although the main materials chemistry challenges, such as ensuring a smooth flow rate, have been solved, large-scale printing is heavy, and the printed parts often weigh thousands of kilograms, making transport difficult. If the MDF project succeeds, transport is a problem that won’t exist, and could see us living in fantastic homes of our own design – from looping mazes to giant statues – with the only limits structural integrity and our imagination.
Welds apart
While the lab’s creations are impressive, much of the MDF’s work is on overcoming the materials challenges associated with such large-scale creations – which falls back to the chemistry of the materials. Plotkowski has a PhD in materials engineering from Purdue University in Indiana, US, with his current research focused on heat transfer and fluid mechanics in materials. ‘In high speed solidification the structures we get are fascinating. 3D printing is just so crazy and new, there’s so many interesting problems that come out of it.’
Metallic 3D printing has been around almost as long as plastics extrusion, and the range of properties parts require mean that the choice of feedstock plays as much a part as the shape, with each metal posing its own problems. One of the facility’s recent achievements was working on aluminium alloys in high-temperature applications. ‘Aluminium alloys have a tendency to be very easy to crack during solidification,’ Plotkowski explains. ‘Even casting most aluminium alloys is pretty challenging. When you weld those alloys, it gets even harder. And metal additive manufacturing is essentially doing thousands of micro-welds in order to create a part.’
The versatility of 3D printing, however, means that the team can always consider different alloys that can overcome conventional shortfalls. In regular casting, metals cool slowly, requiring heat treatments or post-processing to improve the alloy’s microstructure. ‘In additive manufacturing the cooling rates are extremely high,’ Plotkowski says. ‘So I can use chemistry to design an alloy that takes advantage of that. The microstructure looks dramatically different, but we still get great mechanical properties.’
We’ve been researching high-temperature nickel superalloys designed to go into jet engines and gas turbines
Manipulating metallic structures at this level has opened up entirely new avenues for creating objects. As a demonstration, the MDF has a small block of a nickel-based superalloy. ‘We can manipulate the process to drive to different microstructures, meaning I can put in different properties, on demand, wherever I want in a material,’ Plotkowski says.
Despite this control, the nature of printing still poses hurdles. Metallic printing may take place at thousands of degrees Celsius, laser and electron beams liquefy the substrate and feedstock, which can be delivered in layers or from an inert gas jet. This creates intense temperature gradients and thermal expansion that can create different stresses each time a part is printed – leading to an unacceptable level of variation. As a result, the Oak Ridge team have installed thermal cameras, allowing them to take images of the thermal field inside their machines. The aim is not just to identify where possible stresses happen but to program the printers to make corrections in real time, bringing a level of reproducibility in microstructures that was previously unattainable. ‘We’ve been researching high-temperature nickel superalloys designed to go into jet engines and gas turbines,’ Plotkowski says as an example. ‘The alloy is very crack-prone, so we’ve worked on trying to optimise the process to get rid of those defects. We’ve now done about 80 of them, and they’re going to be put into gas turbines and tested.’
The team is also focusing on plastics. Currently, the most common liquid binder used to cohere deposited material is furan (C4H4O), which is poorly soluble in water, a toxic waste product and leaves a rough trace from the mould that prevents highly detailed casting. Instead, an MDF team led by researchers Tomonori Saito and Amy Elliot have been developing novel high-strength liquid binders that are water-soluble and allow for sharper textures than furans, allowing the team to create intricate custom moulds that can then be washed away. This means the team can produce more complex, detailed pieces in a technique that is also more environmentally friendly than standard 3D prints. The binder, developed in collaboration with ExOne, was listed as one of the top 100 innovations of 2019 by R&D World magazine.
Material gains
In overcoming the challenges posed by machines and feedstock, the team has also begun to explore even more exciting potential materials. Following on from its research into carbon fibre, the lab wondered whether other materials could be used for construction. ‘We’ve looked at bio-derived materials as well, such as natural cellulosic fibres,’ Plotkowski says. ‘Imagine there’s a natural disaster in Puerto Rico and you want to print telephone poles. Well, something that Puerto Rico has in abundance is bamboo, it’s a perfect climate for growing that. So we can send down a 3D printer and they can make those fibres on site [out of bamboo], mix it with whatever low cost polymer they like in the right proportions, and then print whatever they need onsite.’
The humanitarian implications could be huge: rather than crates of aid with a multitude of supplies taking weeks to coordinate, disaster relief would need a single 3D printer to mass-produce whatever is required at the disaster hotspot. While such rapid printing isn’t going to produce a smooth, perfectly tooled part, such luxuries aren’t necessary when lives are at stake. ‘We’re doing some work on that right now, trying to understand finishing processes,’ Plotkowski says. ‘There are some critical surfaces that do have to be machined to an extremely high precision – because it’s going to affect fluid dynamics, engine efficiency or combustion – while there are others where it’s not as important.’ Elsewhere at Oak Ridge, teams are investigating how bio-derived materials such as nanocellulose and lignin can be used for printing feedstock.
Complexity is less expensive than it used to be – it’s easier but it’s not free
And yet even the most enthusiastic fans of 3D printing acknowledge that it’s unlikely to result in creating anything in an instant, as imagined by the likes of Star Trek. ‘I can’t just make anything that I want to and it costs me nothing,’ Plotkowski says. ‘Complexity is just less expensive than it used to be. It’s easier but it is not free, and you don’t just get anything that you want – although we can get pretty close. Really, the question is maturing the technology to the point where industry sees those unique advantages.’ Plotkowski pauses for a moment and grins. ‘And, by the time industry really invests in a project, we will probably be moving on to whatever comes next…’
Kit Chapman is a science writer based in Southampton, UK
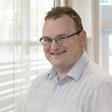
No comments yet