Jamie Durrani speaks to researchers exploring how epigenetic changes in the brain affect drug-seeking behaviours
Each year, one in every 17 people between the ages of 15 and 64 will use a recreational drug at least once. Worldwide, this works out at almost 300 million people. Around 40 million of these people are affected by substance-use disorders, where occasional use has evolved into a much more serious habit.
These conditions are characterised by patterns of behaviour that see people taking a drug, increasing the dosage and then continuing to take the drug despite adverse effects to their health, wellness and lifestyle. In many cases when people try to break these habits, their cravings worsen over time and lead to almost inevitable relapses.
There are many reasons why people might start using drugs and a whole host of factors that play a role in their susceptibility to addiction. But one intense field of research is attempting to unravel whether chemical changes that take place in the brain after someone takes a drug could explain why they will then go on to prioritise seeking out that drug, even if it comes at a heavy price to their own health and wellbeing. The hope is that understanding the roles of certain genes and proteins in particular cell types can aid the development of therapeutics that reduce cravings, potentially offering new ways to treat addiction.
Hijacking the reward circuit
In simplistic terms, addictive drugs can be considered to hijack the brain’s reward circuit – the parts of the brain that have evolved to mediate responses to natural rewards like food, sex and positive social interactions. At its core, this is due to the way in which drugs disrupt the normal operation of dopamine-releasing neurons and other cells in these brain regions.
‘When neurons are activated in the brain, one of the things they do is change how genes are expressed in cells that are activated – this is not specific to drugs of abuse, but it’s a mechanism that drugs engage,’ explains neurobiologist Jeremy Day, from the University of Alabama at Birmingham, US. When a neuron’s dopamine receptors are activated, signals are triggered that ultimately engage the cell’s nucleus, where – crucially – DNA is stored. It’s at this point that epigenetic mechanisms, essentially chemical switches, begin toggling different genes on and off.
To understand what’s happening here, it’s helpful to have a picture of how DNA is packaged inside a cell’s nucleus. We’re all familiar with DNA’s double helix, the long spiral staircase on which our genome is encoded. But within a cell’s nucleus, DNA itself actually wrapped around proteins called histones, and packaged into little balls known as chromatin, chains of which make up our chromosomes. How tightly these chromatin parcels are wound dictates how easily a cell’s machinery can access certain stretches of our DNA. This affects how likely it is that different genes will be transcribed into proteins – a key process in differentiating cell types and determining how they will function.
It’s kind of curious that the first thing the cell does is turn on genes that turn on other genes
Some enzymes can add chemical tags to chromatin, either causing it to loosen up, making genes more accessible, or causing it to wind up more tightly and lock away the stretches of DNA that code for particular proteins. Acetyl groups, for example, attract proteins that help to unfurl chromatin causing the genes in these regions to become activated. Methyl groups can cause chromatin to stay more tightly bound, deactivating particular genes.
Another epigenetic mechanism by which genes are regulated involves proteins called transcription factors. These molecules bind to specific stretches of DNA and either promote or block the activity of RNA polymerase – the enzyme that reads genetic information and produces RNA molecules that guide the synthesis of proteins.
In recent years, scientists have shown that these epigenetic processes are an important feature of many diseases, and our growing understanding of this is promising to transform the treatment of various illnesses. In the case of addiction, it is believed that long-lasting epigenetic changes to the activity of certain genes, and thus the expression of many proteins, helps to drive drug-seeking behaviour – offering an explanation for why users might still feel strong cravings for a drug many weeks, months or even years after the last time they took it.
Day explains that in response to exposure to a drug, the genes within a neuron are activated in two waves. The first of these waves activates genes that code for transcription factors. ‘It’s kind of curious that the first thing the cell does is turn on genes that turn on other genes,’ he says.
‘So the first wave is transcription factors, and it’s going to influence the second wave of genes. And the second wave is things like receptors, ion channels, neuropeptides and signalling molecules that actually influence how the neuron functions,’ he adds. ‘So you go from changing something that just changes other genes to now changing things that actually matter for what the neuron is doing on a minute-by-minute basis.’
Much of our knowledge of how these processes influence drug-seeking behaviour has been gained through rodent models, although studies in other animals and analysis of human brain tissue suggest that closely related molecular mechanisms are conserved across different species.
Early clues
Neuroscientist Eric Nestler is the director of the Friedman Brain Institute at the Icahn School of Medicine at Mount Sinai, New York. For almost 40 years, Nestler’s lab has been trying to unpick how repeated exposure to drugs brings about chemical changes in the brain that mediate the long-term behavioural traits associated with addiction.
‘Early on, one of the major conclusions was that all drugs of abuse – despite very different initial actions – do converge at producing some of the same chronic changes in the brain,’ says Nestler.
A major strand of Nestler’s research has focused on a transcription factor known as ΔFosB. Fos proteins are a collection of structurally related molecules that are rapidly expressed in the brain after administration of a drug. But unlike its family members, which are metabolised within a few hours, ΔFosB is remarkably stable and persists in the brain for a much longer period of time.
Nestler’s team has shown that in response to repeated exposure to almost any recreational drug, ΔFosB accumulates in cells in a part of the brain called the nucleus accumbens – a key part of the reward circuit. The build-up of this protein triggers a whole cascade of downstream molecular signals that seem to play crucial roles in addiction.
‘ΔFosB was a particularly interesting example because we had shown in those early years in my lab that essentially every class of drug of abuse induces ΔFosB in one nerve cell type in one brain region of a mouse and rat brain. And we even validated that in human postmortem brain tissue,’ says Nestler. ‘So that was an important finding: there is something common to all of these drugs, despite their very different chemical structures and the proteins with which the drugs interact initially.’
Creb and Rheb
Another transcription factor called Creb was also identified early on as contributing to addiction-like behaviour. Creb is activated in cells in many brain regions in response to opiates and is linked to several aspects of tolerance and dependence. In the nucleus accumbens, Creb is activated not only by opiates, but also stimulants like cocaine and amphetamine.
Nestler explains that while ΔFosB increases an animal’s interest in consuming rewards like food and drugs and produces a positive emotional state, Creb mediates a more aversive emotional condition – but one that ultimately also drives drug-seeking behaviour. ‘ΔFosB causes an animal to take more drugs because it feels good, it wants the positive experience – it’s called positive reinforcement. Whereas Creb would cause an animal to take more drug because it wants to treat this negative emotional state – so-called negative reinforcement,’ he says. ‘And we know that in people with addiction syndromes, both of those factors are at play.’
Interestingly, while ΔFosB and Creb are implicated in the actions of many drug types, Nestler’s lab has recently identified subtle differences in how these changes occur in response to different drugs. 95% of the nerve cells in the nucleus accumbens are a type called medium spiny neurons. These are divided into two subtypes based on the type of dopamine receptor that they express – half of the cells express a D1 receptor, while the other half express the D2 receptor. The two cell types differ with respect to the inputs they receive and the outputs that they send to other parts of the brain.
While all addictive drugs induce ΔFosB in the D1 medium spiny neurons, certain drug classes differ in their effects on the D2 subtype. ‘Opioids converge a lot in producing many of the same effects as cocaine on D1 cells, but in addition, produce a whole additional set of long-term changes in the D2 cells,’ explains Nestler.
While studying this phenomenon, Nestler’s lab discovered that a gene called Rheb, which is involved in many cell processes, is correlated with Fos expression brought on by exposure to both cocaine and morphine. After using Crispr tools to knock out the Rheb gene in mice, the researchers found that drug exposure no longer reduced the rodents’ desire to seek out natural rewards like food and water. Nestler points out that this is one of the first times that a neural substrate has been identified that appears to play a direct role in shifting behavioural priorities away from essential health-related activities towards drug-seeking. ‘People say the drugs hijack the natural reward circuits in the brain – this is one of the first demonstrations of that happening,’ he adds.
A multitude of mechanisms
As technology has evolved, researchers have discovered many more proteins that appear to be associated with addiction. Modern sequencing approaches have allowed researchers to analyse chemical changes in cells without bias: rather than designing experiments to monitor particular proteins that are already implicated in drug reward, these new open-ended approaches have allowed researchers to carry out genome-wide analyses of cell responses to drug exposure.
‘There are two main conclusions from these more recent studies,’ says Nestler. ‘One is that it was really encouraging, because the data did implicate Creb and ΔFosB, so that was really nice – it meant that the early hunches, the insight we got from those early proteomic studies, did put us on a good track, because we found molecules that really do seem important at a genome-wide level,’ he explains.
‘But having said that, ΔFosB and Creb are really just two of a relatively large number, perhaps 10 or so, key transcription factors that are driving the same types of changes in the nucleus accumbens and other brain regions,’ he adds. ‘And that, in turn, has led us to study many other transcriptional mechanisms, and ultimately to look at the various transcriptional mechanisms for possible therapeutics.’
In Alabama, Day’s lab has used cutting-edge sequencing technology to analyse drug-induced gene changes at the cellular level. In 2020, the group applied single-cell RNA sequencing to understand which genes are up- or down-regulated in different cell types in the nucleus accumbens of rats within the first hour of cocaine exposure. The project confirmed that D1 medium spiny neurons are the cell type that display the most transcriptional level changes.
‘What was additionally surprising was that the cell type that experience the next largest number of changes were actually astrocytes,’ says Day. ‘This is a non-neuronal cell type, which some emerging work has started to identify may also be responsive to drugs as well.’
Day notes that the changes that take place in neurons and in non-neuronal cells relate to a very different set of genes. ‘Previously, if we just took all the tissue together – even if we had genome-wide sequencing approaches – we would get a list but we wouldn’t know where individual genes were changing in which cell types,’ he explains. ‘So this really told us for the first time where to look for specific types of changes, and dissociated for us the changes that are happening in the neurons and the changes that are happening in non-neuronal cell types.’
We were able to artificially increase their sensitisation to cocaine just by turning on this core set of genes
To understand how the early wave of transcriptional changes programmes a neuron’s longer-term response to drug exposure, Day’s team used Crispr tools to target 16 key genes identified in the sequencing study. ‘We could artificially turn these genes on in the absence of drug exposure, and then ask how that changes the molecular makeup of the cells,’ he explains. ‘It turns out that it dramatically changes it – just turning on those 16 genes changes thousands of additional genes. So these are really the key regulators that influence some of these downstream changes.’
To find out what these gene changes mean in the context of drug-seeking behaviour, Day’s team used the Crispr tools to activate the initial 16-gene programme in rats that had never been exposed to cocaine. When then given very small doses of the drug, these rats displayed behaviours that would be expected in animals that already been repeatedly exposed to the drug.
‘We were able to artificially increase their sensitisation to cocaine just by turning on this core set of genes that cocaine normally induces,’ says Day. ‘So at the molecular level, we can bypass what the drug is doing – we can just make these changes and it looks like the animal has already been exposed to the drug.’
Alternative splicing
It’s not just which genes are turned on or off that plays a role in addiction. Elizabeth Heller, a pharmacologist at the University of Pennsylvania, US, explains that individual genes themselves can actually code for different proteins – a phenomenon known as alternative splicing, which also appears to influence drug-seeking behaviour. ‘We learned a long time ago that one gene can make many different proteins, because it has exons and it can splice and dice those exons together in different patterns,’ she says. ‘Those different patterns make RNA isoforms, or protein isoforms. And they can functionally be very, very different.’
Through this mechanism drugs like cocaine actually induce more changes in the protein isoforms that a cell produces, than in the quantities of proteins that are expressed by up- and down-regulation of different genes. According to Heller, these changes in protein splicing represent an underappreciated but crucial factor in how the brain responds to stimuli of all kinds.
Now we’re looking for RNA binding proteins that might interact with that epigenetic change
In 2021, Heller’s team showed that one specific histone modification caused by cocaine exposure shifts the ratio of isoforms of a protein called SRSF11 that are produced in nucleus accumbens neurons. Using epigenetic editing tools, Heller and her colleagues were able to recreate the histone modification in mice, bringing about the same change in the splicing pattern of the SRSF11 protein. ‘It turns out when we alter the splicing of SRSF11 epigenetically, we also increase drug reward behaviour,’ she explains.
Heller’s lab is now trying to unpick exactly how the histone modification changes the splicing patterns. ‘Histone mods don’t cause splicing, much the same way they don’t change gene expression – there has to be engagement of RNA binding proteins. Which ones?’ she says. ‘So now we’re looking for RNA binding proteins that might interact with that epigenetic change, to actually affect the change in splicing.’
Sex-dependent mechanisms
Another recent finding that has complicated the picture of how drugs influence our brain’s chemistry relates to biological sex. Nestler explains that historically, most labs focused solely on male rodents and male humans in studies of addiction.
‘That began to change several decades ago for clinical studies, when government agencies required inclusion of women in trials. But the basic [research] world remained focused on male animals until 10 or so years ago when NIH and other government agencies around the world basically said: “Listen, you need to consider sex as a biological variable,”’ he explains. ‘And I think that turned out to be an extremely important and correct decision, not only from a societal point of view, but from a scientific point of view, because what has been discovered over the past decade are vast sex differences that really had been underappreciated through this time.’
The way that males and females get there isn’t always exactly the same, but the outcome oftentimes will be pretty similar
In 2021, a team led by Vanderbilt University’s Erin Calipari showed that self-administration of cocaine over ten days significantly changes the expression of 82 proteins in the nucleus accumbens of male mice, whereas only 50 proteins were impacted to a similar extent in female mice. Across all of these proteins, only five were altered in both sexes and only three of those were regulated in the same direction.
These dramatic differences reflect the various ways in which neurons can respond to an increase of dopamine in the system, explains Calipari, who serves as the director of Vanderbilt’s Centre for Addiction Research in Tennessee, US. ‘You can reduce the amount of dopamine you make, you can reduce how much is released, you can reduce how sensitive the release is to incoming signal, you can reduce the number of receptors postsynaptically,’ she explains. ‘And so I think what ends up happening … is that the way that males and females get there isn’t always exactly the same, but the outcome oftentimes will be pretty similar.’
‘You see similar changes in males and females over drug use: they take more drug over time, they show drug-seeking behaviour, they show increased responsivity to cues, they’ll show increased dopamine release to drug-associated cues,’ she adds. ‘And so all of this is happening and if you look across those measures, they don’t look that different. But if you look at the molecular mechanisms that seems to be underlying these, they’re different in a way – but the outcome is the same. And so I think this complicates how you target these systems, or what you choose to target.’
Calipari points out that the findings raise new questions about how exactly hormones influence addiction. ‘One thing that’s really kind of cool about hormones is that they’re cyclical. But when you’re on a [birth control] medication, they’re not,’ she says. ‘And so the question around hormonal regulation of addiction … becomes: is it just whether the hormones are there or not? Or is it that they’re cyclical, and that’s priming different systems? I think that’s really interesting.’
Recovery and potential new treatments
Epigenetic mechanisms aren’t just linked to the onset of addiction, but are also involved in how the brain attempts to reset itself during periods of abstinence. Heller’s lab has shown that within one day of withholding cocaine from mice that had previously received daily doses, a Creb-linked transcription factor called NR4A1 becomes activated in neurons in the nucleus accumbens.
One of NR4A1’s targets is a gene called CartPT, which codes for a neuropeptide that helps to regulate dopamine metabolism. Hellers’ team showed that it takes a month for NR4A1 enrichment and the removal of repressive histone modifications to fully activate the CartPT gene. However, when Crispr tools are used to activate NR4A1 in mice, they display a significant reduction in cocaine-seeking behaviour in comparison to control groups.
Heller explains that the finding is even more promising as NR4A1 is a druggable protein. ‘It’s a nuclear transcription factor, which are ligand binding molecules that regulate transcription after binding a ligand,’ she says. ‘There might not be an endogenous ligand for NR4A1 – no one’s found one – but there are small molecules, even naturally occurring ones, that will activate it.’
Heller’s team showed that administering a compound called cytosporone-B, which activates NR4A1, inhibits drug reward behaviour in mice. The team are now investigating other small molecules as well as the epigenetics of CartPT to try to gain new insights into ways of treating addiction-linked behaviours.
Nestler’s team are also collaborating with medicinal chemists at the University of Texas to develop small molecules that target ΔFosB. In 2022, the team showed that thiol-containing compounds can selectively target the protein by covalently binding to one of its cysteine residues. The work is also at an early stage, but points a way forward for future drug discovery efforts to target the protein.
Meanwhile at Vanderbilt, Calipari notes that research is underway at the university’s Warren Center for Neuroscience Drug Discovery to develop allosteric modulators of neuronal receptors that change their function rather than activating them directly like a traditional ligand.
Dopamine is really not a reward molecule at all – it just helps us learn really important information
One difficulty when designing drugs that alter dopamine regulation is that dopamine is involved in all sorts of biological processes. ‘We call it the reward molecule or the pleasure molecule. But … it’s really not a reward molecule at all – it just helps us learn really important information,’ explains Calipari. ‘And so if you put your hand on a hot stove dopamine goes up just as much as it does when you have something pleasurable.’
‘If you block that, people can’t learn, they can’t move – because dopamine is involved in motor – they don’t have motivation,’ she adds. ‘And so it’s a really complicated system where we have a lot of understanding of the different molecular mechanisms, but all those molecular mechanisms are things that we need for just normal biological function.’
Although fundamental research into the brain chemistry of substance-use disorders might still be a long way from delivering new treatments, Heller believes that the growing interest in epigenetic editing offers a promising path forward.
‘We’re already seeing epigenetic editors coming out as putative drugs going into clinical trial,’ she says. ‘There are eight companies right now – Sangamo is one of them and Epic Bio is another one – that have identified epigenetic targets in, for example, neurodevelopmental disorder and a lot of neurodegenerative disorders.’
‘So I think that the feasibility for epigenetic editing for psychiatric disorders is stronger now, because epigenetic editing in general is moving into the clinical space,’ she adds. ‘The challenge is the targets affect so many different genes. So how do we make it specific? If we target one gene, will that be sufficient? If we target a gene that affects other genes, will that be too much – will we get undesirable side effects?’ Despite the complexity of this challenge, Heller is optimistic. ‘I think we’re going to figure it out,’ she says. ‘And maybe now is the time in the next 10 years with these epi tools.’
Jamie Durrani is senior science correspondent at Chemistry World
For a longer version of this article, including discussion of differences between the sexes in this area, please visit LINK
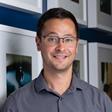
No comments yet