Julia Robinson explains how quantum dots went from a theoretical prediction to everyday reality and earned their creators the 2023 Nobel prize in chemistry
When Alexei Ekimov, Louis Brus and Moungi Bawendi carried out their first work on quantum dots over 30 years ago, none of them anticipated the enormous impact these tiny crystals would have.
Their work, alongside others, was part of the birth of nanoscience and nanotechnology. And while quantum dots already have an array of applications – from QLED television displays to medical imaging – we are still just scratching the surface of their full potential.
They really did something creative and unique
‘When you stop to think about it, it was a fundamental scientific development that has genuinely led to commercial products and much excitement about what could come in the future within a relatively, in scientific terms, short period of time,’ says Euan Kay, an organic chemist based at the University of St Andrews, UK.
‘The clarity with which we understand these systems is a direct product of [Ekimov, Brus and Bawendi’s] intellect, their commitment, and the way that they built the community around these materials,’ says Jill Millstone, an inorganic and materials chemist at the University of Pittsburgh, US. ‘They really did something creative and unique; the field is huge and feels infinite in its possibility.’
In recognition of this, on 4 October 2023, Ekimov, Brus and Bawendi were jointly awarded the Nobel Prize in chemistry for the discovery and synthesis of quantum dots.
But their progress to get to that point was far from straightforward. It was many years after the nanoparticles were first discovered that they were able to be used, both in the lab and in commercial devices.
Quantum properties
Given their name in 1986 by physicist Mark Reed, quantum dots are nanometre-size crystals with semiconducting properties made of combinations of transition metals and non-metal or metalloid elements, most commonly cadmium selenide and cadmium telluride.
As they are so small – just 2–10nm in diameter – a quantum dot’s electrons experience quantum confinement in all three spatial dimensions. Changing the size of a quantum dot changes its properties, in particular its fluorescence, meaning that they can be tuned to different colours. For example, the smallest dots will emit more shorter-wavelength blue light than longer-wavelength red light.
The principle of quantum confinement, also referred to as the ‘particle-in-a-box’ problem, was theoretically proposed in the 1930s when physicist Herbert Fröhlich pioneered the concept that material properties can depend on the macroscopic dimensions of a small particle.
Fröhlich explored the consequences of the Schrödinger equation, which shows that when particles become extremely small there is less space for the material’s electrons and, in turn, the electrons are squeezed together. In the decades that followed Fröhlich’s work, researchers presented further studies of quantum size effects.
In the 1970s, researchers succeeded in making nanostructures and demonstrating that their optical properties indeed varied depending on size – but their observations were related to structures on top of bulk materials, which could not be considered materials by themselves. Almost 50 years after Fröhlich’s predictions, they were still not yet accessible to experimental test.
‘Quantum dots was the most impressive and robust manifestation of the idea of quantum confinement; the idea that when you go smaller than the wave function of the electron, you’re going to start seeing properties of material change,’ explains Omer Yaffe, a materials chemist at the Weizmann Institute of Science in Israel, who was a member of Brus’ lab between 2013–2016. ‘[But] demonstrating it experimentally was a whole different ballgame.’
Quantum dots in glass
In 1979, Ekimov, a solid-state physicist working at the Vavilov State Optical Institute in Soviet Russia alongside theoretician Alexander Efros, began studying semiconductor-activated glasses, known as Schott glasses, and developing theories to explain their colour.
The technology of colloidal particle growth was developed during the Middle Ages to provide the stained glasses that were used in the windows of medieval churches: the variety of colours found in stained glass are due to the homogeneously distributed inclusion of small particles of some metals, metal oxides, and even semiconductors.
Ekimov wanted to determine the structure and chemical composition of colloidal particles in coloured glasses and their mechanism of growth, so decided to study the effect of glass activation by a single compound. The first few experiments were largely unsuccessful but then they came to study glass activated by copper chloride.
The glass was heated to a range of temperatures between 500°C and 700°C, with the heating time varying from 1 hour to 96 hours. Once the glass had cooled and hardened, x-rays revealed that tiny crystals of copper chloride had formed inside the glass. They also found that the exact temperature and duration of heat treatment affected the average size of the crystals that formed: in some of the glass samples they were only about 2nm, in others they were up to 30nm.
Most importantly, Ekimov and Efros found that the light absorption of the glass was affected by the size of the particles; the bigger particles absorbed the light in the same way as bulk copper chloride, while the smaller particles absorbed bluer light. As a physicist, Ekimov immediately understood that what they had observed was a quantum size effect.
This was the first time someone had succeeded in deliberately producing quantum dots, nanoparticles that cause size-dependent quantum effects. Ekimov published the findings in a Soviet scientific journal in 1981. One limitation of his groundbreaking discovery was that the quantum dots his team had found were ‘frozen’ in glass and it was not possible to use them in any way other than to study their optical properties.
Finding a solution
Around the same time but separated by distance and the Iron Curtain, Louis Brus at Bell Laboratories in New Jersey, US, was studying organic photochemistry on cadmium sulfide particle surfaces using pump–probe Raman spectroscopy. His aim was to make chemical reactions happen using solar energy and was using particles of cadmium sulfide, which can capture light and use its energy, to drive reactions.
During this work, Brus observed quantum size effects almost accidentally when he noticed that the optical properties of the cadmium sulfide crystallites changed after he had left them on the lab bench for a day.
‘I noticed by chance that the band gap appeared to be a function of size,’ Brus explains. ‘Some days when I made the very small particles for the first time, the band gap was larger… and if you just let the colloid sit, Ostwald ripening would occur, and then it would grow to larger sizes, and the band gap would become the normal band gap.’
Brus attributed this difference between small and large particles to a quantum size effect moderated by electrostatic interactions between electron and hole. After he realised what was going on he switched his research so that he could focus entirely on these ‘little particles’; and extended his studies to zinc sulfide and selenide, cadmium selenide and the silver halides.
‘Solid state physics is not my field, but over the weeks it dawned on me that this was important because what’s at stake here is this transition between molecular behaviour for small molecules and solid state behaviour for larger crystals and that was not really understood – chemists understand molecules and physicists understand the bulk crystals – but it was going to become relevant,’ he explains.
Brus published his discovery in 1983 but it was not until the following year that he learned of Ekimov and Efros’ efforts when he read translations of their paper.
‘He was behind the Iron Curtain and he published in Russian; nobody read those rather obscure Russian journals. In the beginning, I asked my friends “Has anyone worked on small particles?” And they said no – his work was not known.’
On finding the paper, Brus sent a handwritten letter to Russia to tell Ekimov and Efros about his own work but it was a while until he heard anything back. ‘Efros told me recently that when my letter arrived in the Soviet Union, the KGB came to talk to them, because why would they have any contact with Western scientists?’ says Brus.
The trio were finally able to meet several years later in the 1990s when Ekimov and Efros obtained exit visas to visit Brus in Bell Laboratories. ‘He very clearly saw these effects working more or less by himself and under conditions that were relatively primitive compared with what we had in the West at that time,’ says Brus.
The final piece of the puzzle
Brus and Ekimov’s discoveries of quantum size effects in colloidal nanocrystals were incredibly significant but there was still a problem to be solved. The methods demonstrated thus far had created quantum dots of unpredictable quality and size, often with defects; a reliable and consistent synthesis method was needed.
‘We struggled to get this colloidal synthesis under control because it was a kind of a black magic in the beginning; it was just a recipe,’ Brus recalls.
It then became a big collaborative effort, involving Paul Alivisatos, who joined Brus’ lab in the 1980s, and Brus’ good friend Mike Steigerwald, who all worked side-by-side to try out different ideas using a range of solvents, temperatures and techniques.
‘The culture of the institution was such that people were supposed to help each other and if you had a question, you could go knock on the door of someone who was a real expert on the subject…and that worked out well,’ says Brus.
Bawendi, who had a background in theoretical physics, spent a summer at Brus’ labs in 1987 as part of a fellowship. That was the first time he met Brus, Alivisatos and Steigerwald, and the first time he encountered the world of quantum dots.
‘It was just amazing to be there with those three people as a graduate student,’ says Bawendi. ‘And so when it came time for me to do a postdoc, I applied to Bell Labs… It was an amazing place, the quintessential interdisciplinary atmosphere … we talked about science all the time.’
At that time Bawendi had never done any inorganic chemistry and until then had not been particularly interested in doing it either. But that all changed when he came to Bell Labs.
‘The work that Louis was doing was basic quantum mechanics – it was so cool. And then Steigerwald allowed me to do some “cooking” [and] to play with chemicals in his lab and that was just great because I learned that I actually liked to do chemistry.’
The magic flask
When Bawendi returned to Bell Labs 1988 as a postdoc there were a number of ‘puzzles’ that Brus still didn’t understand about some of the particles they were making.
‘So what Louis, Steigerwald and Paul had done together was to make small particles in an inverse micelle medium and they had basically invented the way of getting the particles out of solution in a powder.’ However, the particles they were making were ‘extremely defective’ because the crystal structure had been formed at room temperature.
‘We were searching all the time for better particles, because it was very clear that what we had in the beginning was poorly crystalline and had very wide side distributions and so forth,’ says Brus.
‘They discovered that if you anneal them in something like pyridine at 90°C , or 4-ethyl pyridine at a little higher temperature, then they would get a little bit better. When you made them really small, the spectrum that appeared became extremely sharp, with a wavelength that was always around the same – 410nm,’ says Bawendi.
But what species had they created? It was Bawendi’s job to find out.
‘I went to Steigerwald and said “So pyridine is a Lewis base, what are all the Lewis bases in your lab?” I had a dozen flasks that were cooking away with different Lewis bases and nothing really worked,’ Bawendi recalls. ‘Except there was one flask; the magic flask. People talk about an “aha” moment… that was the “aha” moment.’
At first, the colour in the ‘magic flask’ slowly went away to nothing but then the next day a ‘beautiful orange-ish red colour’ had appeared which fluoresced under UV light. ‘You could see red fluorescence in a way that we had not seen before. I took a spectrum of it and it was this beautiful spectrum – it was so sharp, a beautiful narrow line that we had never seen before,’ Bawendi says.
By that point it was late December and the lab was closed for the holidays, but when Bawendi returned and tried to recreate what he had seen, nothing worked. Puzzled, he went to Steigerwald who suggested that they analyse the bottle of trioctylphosphine surfactant he had used. They realised that when it had worked, the bottle had been sitting on the shelf a while and was no longer what it said on the label: it had oxidised.
It was a very, very special time
‘Once we realised, I bought the oxide [trioctylphosphine oxide] and mixed it in different quantities and I was able to reproduce the [finding]…the issue was I could only make one size, which was great, but I couldn’t tune it.’
When Bawendi left Bell Labs in 1990 to take up an assistant professor position at Massachusetts Institute of Technology, he took the project with him while Brus switched to work on silicon particles. But for around six months, Bawendi had no success.
‘Chris Murray, David Norris and Manoj Nirmal, were my three first students, they were great – I was so lucky to have them,’ says Bawendi.
‘My project was to develop the synthetic methods to make and characterise the semiconductor quantum dot systems that were used in the further study,’ explains Murray, now a professor of chemistry and material science at the University of Pennsylvania in the US.
‘There were three students who came together in that first year as Moungi arrived at MIT; we were just all very excited to get started,’ Murray says. ‘It was a great experience to be part of the first efforts to translate the ideas and the vision that Moungi had coming out of his work together with Louis Brus… it was a very, very special time. The expansion since then has been just beyond all imagination. But that sense of exploration really marked those first years.’
At that time, Bawendi was spending a lot of money buying a continuous supply of trioctylphosphine oxide, until Murray found that the longer chain version was much cheaper and had a higher boiling point, which brought additional benefits.
The hot injection method
Within two years, with further adjustments to their method including switching the precursors – which meant getting rid of the inverse micelle powders – they finally made a breakthrough: hot-injection synthesis.
‘You inject room temperature chemicals into a hot solvent, quickly,’ explains Bawendi. ‘That creates a nucleation event, and then the temperature drops, and that quenches the nucleation.’ The sudden drop in temperature causes any growth to stop, but reheating to the desired temperature allowed the growth to continue in a controlled way by way of Ostwald ripening. The result were cadmium selenide quantum dots with a regular core structure and shape and well-defined size.
‘You could get the whole size series all the way from the really tiny ones with this sharp peak at 410nm, all the way to things that had the band gap of the bulk. And because it’s cadmium selenide, everything happens in the visible from the pale yellow for the very smallest particles to this deep red colour for the biggest particles… you see the quantum mechanics forming in front of your eyes.’
In 1993, Bawendi and his team published their hot injection synthesis method for making quantum dots of high quality and consistent size in the Journal of the American Chemical Society. The method was adaptable and reproducible, meaning it could be used by others wanting to synthesise monodisperse nanoparticles using a wide range of material systems.
In other words, their work finally opened the door for the development of large-scale applications of quantum dots.
‘The beauty of that synthesis is that it’s still now the basis of the vast majority of quantum dot syntheses, even today with all the developments we’ve had in the last 30 years,’ says Kay. ‘It was really a beautiful piece of applying inorganic chemistry, synthetic chemistry, the chemistry of crystallisation and putting that together to develop something that became a really robust synthesis that’s really stood the test of time.’
Mathias Brust, a chemist working on gold nanoparticles at the University of Liverpool in the UK, says the method was ‘the holy grail’. ‘Everybody who was working [in this area] was interested in this effect, it was a step change for them because they now had a method that claimed that you can make any size at will,’ he adds.
For Millstone, the trio’s work goes beyond the synthetic route. ‘It’s not just in making the materials well, but it’s really describing the physical chemistry that they represent, that shows how nanoscale materials in general represent a frontier of unknown physical properties.’
An array of applications
Now quantum dots are the most heavily studied nanoscale semiconductor structures and are found in an ever-growing range of commercial products thanks to their tuneable properties.
‘[The field] exploded,’ says Yaffe. ‘With quantum dots the advantages were that they were semiconducting and all the things you can do with semiconductors – light and electronics – were all there to investigate and study.’
Their applications span biomedicine, catalysis, photovoltaics, photoconductors and photodetectors, but the most well-known applications for most are in televisions and computer screens based on quantum-dot light-emitting diode (QLED) technology. In these screens, blue light is generated using energy-efficient diodes (recognised with the Nobel Prize in Physics 2014) and quantum dots are used to change the colour of some of the blue light, transforming it into red or green. This makes it possible to produce the three primary colours of light needed in a television screen.
It was the electronics company Samsung which principally made the connection between quantum dots and television. ‘Samsung deserves a lot of credit, they put a lot of money into the development of the technology so that they could use them in their own TVs,’ says Brus.
There were technical problems that needed to be resolved; for example, quantum dots based on cadmium needed to be replaced with ones that were highly efficient light emitters but did not contain such toxic heavy metals.
‘Cadmium selenide is not consumer friendly, even if it’s completely protected, you don’t want to be using it,’ says Bawendi. ‘[Samsung] realised that and invested a lot of money in indium phosphide as a replacement to cadmium selenide, which is a harder material to make.’
Quantum dots are also used in some LED lamps to adjust the cold light of the diodes to make them have a warming, more inviting glow. And, in medicine, the high luminescence, narrow emission and biocompatibility of quantum dots make them a perfect candidate for bioimaging, diagnostics and biosensors; biochemists have attached quantum dots to biomolecules to map cells and organs in the body and explored their use in drug delivery, while doctors have begun investigating the potential use of quantum dots to track tumour tissue in the body.
Quantum dot synthesis, characterisation and applications remain a highly active field of investigation. ‘A huge potential application is in photovoltaics as an energy conversion,’ Kay predicts. ‘I think some of the optical properties might lend themselves to more photodetection, sensors, infrared sensing, night vision – those types of applications.’
Bawendi is currently looking at quantum optics applications: ‘There’s a lot of interest in quantum materials, that could be used in things like quantum computing, quantum communication, basically manipulating the quantum mechanical properties of excitations in the material,’ he explains.
‘What we discovered a few years ago was that perovskite-based quantum dots can emit a very special kind of photon. So again, we’re back to the roots to figure out why this works, how it works, and how do we make it better?’
Although it may be some time before we realise the full potential of quantum dots, it is clear these tiny particles have already made a difference to many people’s lives. As Murray says, ‘It certainly is a demonstration that it takes some time for fundamental new insights to translate into the impact that often then captures a wider imagination. The prize recognises that the science that surrounds nanoscience and quantum dots specifically has matured in a way that its impact is being felt by many, many people.’
Julia Robinson is a science correspondent at Chemistry World
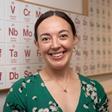
No comments yet