Hydrogen atoms can be selectively extracted from symmetrical molecules using a catalyst readily derived from Cinchona alkaloids, researchers in the UK have shown. The researchers went on to form carbon–carbon bonds using the resulting chiral radicals. They believe the work could potentially have widespread applications in pharmaceuticals and other fields of synthetic chemistry.
Hydrogen atom transfer is a fundamental mechanistic process in chemistry, and enantioselective hydrogen atom transfer from a symmetrical compound can introduce chirality into the product. This can in principle be achieved either by enantioselectively attaching a hydrogen atom to an achiral radical or by selectively removing one of two hydrogen atoms from a symmetrical molecule, creating a chiral molecule that can subsequently form further bonds. Researchers have achieved significant success with the first strategy, but progress with the second has remained elusive.
In 2015, the group of organic chemist David MacMillan at Princeton University in the US – who was to share the 2021 Nobel Prize for work on asymmetric organocatalysis – established that quinuclidine could act as a versatile catalyst for hydrogen atom abstraction adjacent to alcohols, and it has subsequently become widely used. However, MacMillan’s work did not explore the potential to induce chirality during this process. ‘His group had largely moved on [from asymmetric organocatalysis] by that point,’ says Robert Phipps at the University of Cambridge in the UK.
In the new work, Phipps and colleagues examined the ability of chiral versions of quinuclidine, derived from Cinchona alkaloids, to catalyse the desymmetrisation of meso-diols under blue light in conjunction with a photocatalyst. This enabled a highly selective epimerisation process – the interconversion of one type of stereoisomer into the other – following replacement of the hydrogen atom that derives from a thiol. The catalyst, derived by direct hydrogenation of a Cinchona alkaloid, showed poor reactivity and enantioselectivity. ‘We found that, if you swap the hydroxyl group to be a protected amine and you invert the stereocentre, then it becomes a much better catalyst for this process,’ says Phipps. The researchers are now working with computational chemists to explain this.
The team went on to show that, by trapping the chiral radical using electron-deficient olefins, it could form carbon–carbon bonds, making a wide variety of new compounds. Phipps believes the future implications could be widespread. ‘Radical-based chemistry is now very mainstream, be it in pharmaceutical chemistry, materials chemistry or biological applications, and a lot of that is increasingly down to the growing popularity of photoredox catalysis,’ he says. ‘We’re showing a proof of principle that this can be done, so if people use quinuclidine in their method, maybe they might like to think about swapping it out and using the chiral version to see if the selectivity changes. There’s also a possibility that this catalyst could be used for site-selective chemistry as well, because if a molecule has different positions, you might be able to control which position it goes for.’
‘I think this paper is great,’ says organic chemist Todd Hyster at Princeton University. ‘Maybe in product selectivity it doesn’t have high synthetic value as it currently stands – there’s lots of nice ways for making cis and trans diols stereoselectively, but it opens up the concept of being able to do catalyst-controlled hydrogen-atom transfer events… One of the feats in the field that is still quite important is being able to control the chemoselectivity or the regioselectivity of late-stage hydrogen-atom transfer events and I see this as maybe being a really important first step towards being able to do that.’
References
ASK Lahdenperä et al, Science, 2024, 386, 42 (DOI: 10.1126/science.adq8029)
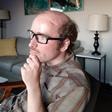
No comments yet