Technologies available now can decarbonise most heat demand, but cost and infrastructure barriers still exist
Supplying the high temperatures needed for industrial processes – like cement, steel and petrochemical production – accounts for around a fifth of total global energy demand. Most of this energy is generated by burning fossil fuels, releasing the equivalent of billions of tonnes of carbon dioxide into the atmosphere each year – roughly one third of humanity’s greenhouse gas emissions.
As such, finding alternative ways to generate industrial process heat is essential as the world attempts to cut emissions and limit the worst effects of climate change. While hydrogen is often touted as a sustainable replacement for fossil fuels, in many cases, the simplest approach could be to use technologies that convert electricity directly into process heat.
Matthias Rehfeldt, an expert on industrial energy demand at the Fraunhofer Institute for Systems and Innovation Research in Karlsruhe, Germany, explains that ‘there is a huge potential for direct electrification’ of many industrial processes. A recent report commissioned by the clean energy thinktank Agora Industry and co-authored by Rehfeldt finds that 62% of industrial process heat in the EU could, in theory, be supplied by direct electrification using technologies that are already on the market. This figure rises to 90% when factoring in technologies that are expected to reach market readiness within the next decade.
As decarbonisation efforts progress, it is likely that a mix of technologies will be required to accommodate the specific needs of different industries. For example, steel or ammonia producers operating at temperatures well in excess of 1000°C will need to adopt different approaches to distilleries or paper mills working in the 100–200°C range. But promisingly, a range of technologies are available that appear ready to meet these vastly different needs.
Lower temperature applications
Rehfeldt points to heat pumps and electric boilers as market-ready technologies that are already able to provide heat for lower temperature processes. ‘The low temperature area is especially interesting, because electrode boilers and heat pumps are available,’ he says. ‘There are some challenges in terms of upscaling … but it’s definitely possible already to do this. And heat pumps, of course, deliver a high efficiency advantage compared to conventional fossil production and that makes them very attractive.’
Heat pump technology has been around since the mid-1800s and transfers heat using the same principles as a refrigerator, by expanding and compressing a refrigerant gas. These systems can recycle waste heat, or source heat from the air, sea or ground and lift it to higher temperatures. Because heat pumps move heat rather than generating it, they can provide much higher amounts of heat output than they consume in terms of electricity input – offering a major efficiency advantage over other technologies. Industrial units can typically provide pressurised steam at temperatures in the 100–300°C range.
MAN Energy Solutions is a leading manufacturer of industrial heat pumps that for decades has supplied systems that recover waste heat from processes like propane dehydrogenation and fluid catalytic cracking at major chemical refineries. Thomas Staude, who leads on industrial heat pump sales at MAN, explains that in recent years the temperatures, pressures and power output that the technology can supply has dramatically increased. ‘Our portfolio of compressors is referenced up to over 65MW electric per casing – so for big plants, big steam production requirements, you can easily go up to 120–150MW thermal units,’ he says.
‘The compressor is not the bottleneck anymore for heat pumps as it was in the past,’ adds Staude. ‘And neither is the temperature that you can produce with this type of technology … So the limitations now are really the handling of these big heat exchangers, how you can integrate it, logistics – but not the core machinery technology or the heart of the heat pump itself.’
Increasingly, the company has been supplying purpose-built heat pumps that can serve as the main source of process heat for industrial-scale operations. One example is a 5MW heat pump used to provide pressurised steam for Absolut Vodka’s ‘carbon neutral’ distillery in Åhus, Sweden. In general, the use of heat pumps in these projects is planned from the outset. However, Staude says that the ‘next challenge’ is to retrofit the systems into existing plants.
While installing heat pumps at pre-existing facilities is technically complex, Staude notes that MAN has successfully completed a feasibility study on integrating new heat pumps into the steam network at BASF’s chemical refinery complex in Ludwigshafen, Germany. This would potentially supply up to 150 tonnes of steam per hour, reducing carbon dioxide emissions from the site by as much as 390,000 tonnes per year. However, Staude says that project appears to be on hold while BASF adjusts to the economic challenges that have impacted Europe’s chemicals industry over the last two years.
Everywhere where you can’t reach with a heat pump – because you need higher temperature – then you would go for electrode boilers
Another retrofit project that is likely to be completed in the nearer term involves a dairy producer in New Zealand. ‘They really want to get rid of their coal boilers and want to provide the process steam with a heat pump, so all signs are pretty positive there,’ says Staude. ‘And then we have a very, very interesting project on the east coast of the US where we are retrofitting a heat pump into an old coal power plant to produce steam for the district heating grid of Boston.’
As another well-established technology, electric boilers also provide an option for processes operating at the lower end of the temperature scale. These can provide superheated steam up to 500°C and come in two forms: resistance boilers that heat water via a heating element, or electrode boilers where current is applied directly through the water itself.
Rehfeldt notes that electrode boilers are the more suitable option for higher capacity industrial systems. ‘We talked with one manufacturer and the usual shift from electric to electrode boilers is at around 10MW – and so on industrial scale, we’re talking about electrode boilers, basically,’ he says. However, boilers don’t have the efficiency advantage of heat pumps – all the energy to heat the water needs to come from the electricity supplied. ‘So I would say, everywhere where you can’t reach with a heat pump – because you need higher temperature or the temperature lift is too high – then you would go for electrode boilers.’
Last year, UPM announced that it would install eight electrode boilers made by Norwegian manufacturer Parat Halvorsen across its paper mills in Germany and Finland. Electrode boilers installed at the company’s Tervasaari site also supply heat for the local district heating network, in addition to the mill itself. According to UPM, the boilers installed at its Finnish sites will cut around 50,000 tonnes of CO2 emissions each year by reducing the need for natural gas.
Getting hotter
For the next range of temperatures from the low hundreds up to around 1000°C, other solutions are required. For these processes, Rehfeldt points to electric resistance heaters as one of the most viable technologies. These systems employ heating elements that either directly or indirectly transfer energy to a material that needs to be heated.
Earlier this year, a pilot demonstration that uses both systems began operation at BASF’s Ludwigshafen refinery. These electric crackers convert long chain hydrocarbons into more useful smaller molecules, producing around four tons of product per hour. BASF says that, when run on 100% renewable electricity, the pilot crackers could cut around 90% of the CO2 emissions linked to the cracking process when compared to existing cracker designs, which release up to two tons of carbon dioxide per ton of ethylene produced.
Resistance heating can also be combined with heat storage systems, where thermal energy is stored in a material like a molten salt, sand or bricks. This energy can then be released on demand to provide hot air or steam that can be used to drive industrial processes. These systems can help to balance fluctuations in heat demand and electricity supply.
Rondo Energy is one of several companies pioneering industrial-scale use of this technology. Its container-sized refractory-brick-based heat batteries can provide hot air or steam heated beyond 1000°C. The company claims that its units store energy with a loss of less than 1% per day.
‘The commercial models that we build are 100 and 300MWh and there’s a larger one as well, that’s still being specified. A 300MWh heat battery delivers 20MW of continuous heat, so it’s about the equivalent of an 85 million BTU an hour boiler,’ says John O’Donnell, Rondo’s founder and chief innovation officer.
‘It’s hot enough to drive cement calcination – we’re building a project right now at a cement plant in Thailand – and to drive all the temperatures used in a petrochemical process from ethylene cracking down through utility steam,’ he adds.
Last March, a 2MWh Rondo unit installed at a bioethanol facility in California became the first electric thermal energy storage system to go into commercial operation in the US. The company has also reached agreements to install units at Diageo distilleries in Kentucky and Illinois that are estimated to eliminate around 17,000 tons of greenhouse gas emissions annually. That partnership is supported with a $75 million grant from the US Department of Energy that was awarded to help Diageo electrify its production sites, with the goal of making them carbon neutral within the next four years. The grant was part of a wider $6 billion effort announced by the US government in March to support 33 decarbonisation projects across energy-intensive industries, which US energy secretary Jennifer Granholm described as ‘the largest investment in industrial decarbonisation in the history of the United States’.
Another project receiving up to $375 million from this funding pot is a new chemical recycling facility for repurposing plastic waste being built in Texas by the Eastman Chemical Company. This facility will use onsite solar power in combination with Rondo’s heat batteries to decarbonise its process heating. The project is expected to produce virgin-quality polyethylene terephthalate (PET) with a 70% lower carbon intensity than fossil virgin PET. Eastman says that PepsiCo has already signed a contract to use materials produced at the facility to create sustainable packaging.
‘We are at this tipping point where intermittent renewable electricity delivered as continuous steam or high temperature heat from a Rondo unit is becoming directly competitive with coal,’ says O’Donnell. ‘And over the next couple of years … as the technology deploys, there are places where the industries are building lots of new infrastructure that can be cost effective and clean, and not be “regret infrastructure” – not be stuff that as soon as we’re building it, we’re trying to figure out how to decommission or change it.’
We are at this tipping point where intermittent renewable electricity, delivered as continuous steam, is becoming competitive with coal
Rondo has also recently confirmed a number of projects in Europe, including a 100MWh system at Covestro’s polymer plant in Brunsbüttel, Germany. The heat battery will supply 10% of the steam required at the site, saving up to 13,000 tons of carbon dioxide emissions annually. Another partnership will see Rondo work with Saudi Aramco to deploy heat batteries up to a scale of 1GWh. O’Donnell notes that the company is working to build experience with different electricity networks as well as construction and finance frameworks in as many different territories as possible, so as to smooth the future deployment of its technology at scale. The company has set itself the ambitious goal of cutting 1% of the world’s total carbon dioxide emissions over the next decade, and a staggering 15% within 15 years.
‘We know the world urgently needs this decarbonisation transition as fast as possible, and we don’t get to making contributions at scale without proving ourselves in those geographies,’ he says. ‘We have projects where the ambient temperature is –60°C and projects where ambient peak temperature is +60°C as part of the design basis. We have projects making almost every commodity across paper, chemicals, fuel refining, food, cement – so we are establishing the foundation now.’
Hitting 3000°C?!
For yet higher temperature applications like steelmaking, Rehfeldt and Agora point to induction heating and electric arc furnaces as existing technologies that are already being applied in industry.
Induction furnaces, which heat metal by means of a rapidly reversing magnetic field, are already used for in some smaller and speciality steel plants. The largest examples were installed around 10 years ago by ABP Induction at a stainless steel facility in Taiyuan, China. These 42MW furnaces can heat up to 65 tonnes of metal to around 3000°C.
Electric arc furnaces can heat material to temperatures up to 1800°C by exposing it to a plasma arc and are increasingly used in scrap-based steelmaking. These are more suitable for larger scale applications, with furnaces providing power of up to 200MW. The world’s largest electric arc furnace is installed at Tokyo Steel’s plant in Tahara, Japan, and can hold 420 tonnes of material.
Recent analysis by Global Energy Monitor (GEM), which tracks the operations of the 1200 biggest iron- and steelmaking facilities around the planet, shows that 93% of planned new steelmaking capacity announced last year will make use of electric arc furnaces. This represents a dramatic shift – of the new steelmaking capacity announced in 2020, just 36% was to be based on electric furnaces.
However, the GEM report notes that less than 14% of the planned electric arc furnaces have moved into the construction phase – meaning that almost half of the projects that are currently under construction are still based on coal-burning blast furnaces.
‘The progress is promising for a green steel transition. Never before has this much lower-emissions steelmaking been in the pipeline,’ noted GEM’s director for heavy industry, Caitlin Swalec. ‘At the same time, the buildout of coal-based capacity is concerning. What the industry needs now is to make these clean development plans a reality, while backing away from coal-based developments.’
Overcoming barriers
Despite the availability of technologies that have the potential to slash industry’s emissions, barriers to their deployment remain. A primary concern surrounds cost. ‘When you look at the entire lifespan of an installation that provides process heat, the total cost of ownership is about 80% dominated by the energy costs – sometimes more,’ notes Rehfeldt. ‘The [capital expenditure] is usually low: maybe 10–15%, but that’s over the entire lifetime – it’s still a challenge to have an intensive [capital] investment because you need to have the capital available, you have a high uncertainty, so you have to weigh your risks. From the individual perspective of a company or production site, that could still be a barrier, but looking at the entire costs, it’s usually not and more the operational costs is the higher challenge.’
With energy costs contributing such a high proportion of the total costs, Rehfeldt points out that transitioning to decarbonised energy sources brings a clear economic challenge. Electricity in general costs more per unit of energy than fossil heat sources. Even accounting for increased efficiency of electrified processes, that can make it hard to make an economic or business case out of switching. ‘That means that the economics somehow have to change,’ he says.
When you electrify you replace your thermal load that you previously supplied with natural gas with electricity, but you need different infrastructure for that
A number of mechanisms can be exploited to make electric-powered processes competitive with existing fossil-based processes, Rehfeldt explains. These include increased carbon pricing to raise the cost of emissions-intensive production, and greater subsidies for electricity-based production, including lower grid fees. ‘There’s also a third aspect … this difference could also be somehow covered by the market,’ adds Rehfeldt. ‘If consumers are willing to pay more for a green product, this [cost] difference could be addressed by that.’
There are also technical challenges for decarbonising industrial heat. Clearly providing enough renewable electricity to supply industrial processes that were previously driven by fossil fuels requires massive deployment of solar, wind and other renewable power. But even once the electricity is available, there can be barriers to bringing electricity-driven processes online.
‘When you electrify you replace your thermal load that you previously supplied with natural gas with electricity, but you need different infrastructure for that. You have the natural gas connection, but these are typically in the area of double-digit megawatts – and some industrial sites only have 5–10MW electricity connections, which they are already using, and so would have to extend the electricity grid connection. That’s something that takes a lot of time. I know that for Germany, it can take four, five years or longer to do this,’ says Rehfeldt. ‘And that means that your window of opportunity for reinvestment is long gone. If you have to wait four years to invest in your core business, then you just invest in the fossil installation and not in the electric one, even if you would have had a business case for it.’
According to O’Donnell, one final challenge surrounds awareness of the different potential solutions to the problem of decarbonising industrial heat. ‘There are plenty of organisations where the chief executive may ask the team: “What’s our hydrogen strategy?”,’ he says. But while hydrogen may have a role in decarbonising certain specific processes, it is likely to be much more expensive and inefficient than direct electrification in most cases. ‘There aren’t that many organisations where they’re asking: “What’s our electric thermal energy storage strategy?” or more broadly, in a technology neutral way, “What’s our clean energy strategy? And what are the pathways?” So awareness is really still the number one problem,’ O’Donnell concludes.
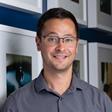
No comments yet