Two new ways to control and optimise electrochemical reactions at electrified interfaces have been discovered. These insights could help to design new catalysts for battery technology and hydrogen production, crucial for the green transition.
Electrolysis involves passing an electric current through a substance to induce chemical reactions, a particularly important process for green hydrogen production and battery technology. However, there is limited understanding of acid–base chemistry at the metal/water interfaces where key steps of electrocatalysis take place. Although it is theorised that acid–base chemistry at the electrochemical interface differs from that in bulk, the relationship between these effects, as well as assessing a key metric – the acid dissociation constant (pKa) values – quantitatively has been difficult.
Scientists from Ruhr University Bochum have now discovered two factors that govern the complex behaviour at electrified gold/water interfaces. A new model to rationalise shifts in the acid dissociation constant values at metal aqueous interfaces has been proposed based on two mechanisms: local hydrophobicity and local electric fields.
The team used surface enhanced Raman spectroscopy (SERS) in combination with modelling to study local and site-specific acid–base chemistry properties of the amino acid glycine. ‘Vibrational spectroscopy is a very good choice because [SERS] is very sensitive and allows you to reliably get the information about the change in protonation state of the two polar groups of the molecule,’ comments lead study author Simone Pezzotti.
‘[Glycine] has two groups that can take or give a proton and when you play with it, you can see that it’s not even a question of local pH,’ says Pezzotti. Compared with the known pKa values of glycine in bulk, the pKa value of both carboxyl and amino groups at the gold/water interface is shifted towards neutrality – thus, the pH window for the existence of the glycine zwitterion is reduced. Simulations confirmed that the two charged groups are partially desolvated at both gold/water and air/water interfaces.
‘From these simulations, we could understand why this zwitterion shrinks and the reason is that at the interface, even though gold interacts very well with water, we do have hydrophobicity.’ A hydrophobic water interface very close to the metal destabilises the zwitterion – it becomes like mixing water and oil. The local pH value at a hydrophobic interface provides a very different solvation environment to the bulk one. ‘This provides a direct quantitative measure of how much less good as a solvent water is when it’s close to a surface than when it is far away,’ explains Ian McCrum, an electrochemistry researcher at Clarkson University in the US, who wasn’t involved with the work.
This was not the only outcome observed – the reduced stability of the zwitterion becomes amplified with increasing applied potential. The local electric field (the sum of the fields from the electrified gold surface, as well as the interfacial water) can either stabilise or destabilise a bond. Electric fields play a significant role as they can catalyse chemical reactions in liquid water – breaking and forming bonds. ‘If we fix the pH and we just play with the voltage, we can induce chemistry,’ says Pezzotti, adding that additional local electric fields induce proton transfer on the glycine. The local electric fields drive the protonation of amino groups not only in hydrophobic solutes, but also in hydrophilic ones.
‘I think that [the research] is going to move us in the direction of understanding how solvent and electric field work together to affect reactions occurring at surfaces,’ says McCrum. The results show that acid–base chemistry at electrified interfaces can be tuned by not only variations in hydrophobicity but also by a variation in applied voltage. ‘There are really a lot of opportunities to tune these two contributions and … tune the chemistry,’ adds Pezzotti.
References
S Murke et al, J. Am. Chem. Soc., 2024, DOI: 10.1021/jacs.3c13633
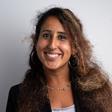
No comments yet