For the first time, researchers have observed communication between the rotors in a molecular motor. Although the exact mechanism is still a mystery, communicating components could convey complex and controlled movements to molecular machines, better mimicking biological systems.
In molecular motors, external stimuli such as light or heat activate movement. In structures with different rotors, usually each rotation operated independently, however here, ‘[we] show that the rotors have a profound impact on each other’, explains first author Carlijn van Beek, who works in the laboratory of Ben Feringa at the University of Groningen in the Netherlands. The communication between the components affects the behaviour of the overall structure. ‘It’s similar to macroscopic gears, [within] a small molecular system,’ says van Beek. ‘The motor [behaves] as two motorised gears – the two rotors – connected via a third gear, the motor core unit.’
To create the motor van Beek combined fragments of previous molecular motors into a new ‘bridged-isoindigo’ structure. According to the authors, the isoindigo structure ‘revealed another dimension to the traditional mechanism of molecular motors’, where a double metastable intermediate connects the two rotor subunits – effectively creating a communication channel. In traditional molecular motors, one component must complete a rotation before the other is activated, she explains. In contrast, the changes in conformation of one rotor in the isoindigo structure somehow sterically ‘pushes’ the central core unit, which then influences the movement of the other rotor. Moreover, it brought some additional advantages, such as increased solubility and a simpler synthetic process. Nevertheless, the design wasn’t straightforward, says van Beek. ‘In molecular machines … achieving the desired function required a delicate balance of different motions,’ she explains.
‘This system is much more complex [than] other systems in the literature,’ says Aisha Bismillah, an expert in supramolecular chemistry at the University of York, UK. ‘It’s designed in such a clever way,’ she adds. ‘Instead of all the parts of the molecule rotating independently of one another, [they] communicate to one another and influence the rotational motion.’ In part, the communication occurs thanks to the carbon–carbon double bonds that connect the rotor ‘gears’ to the central unit. The study is especially interesting, says Bismillah, as the authors went through ‘a lot of detail to show full understanding of how each of the 12 steps [connecting the eight different isomers] work’.
To investigate the intricate mechanism of the molecular motor, van Beek used a combination of NMR and UV-visible spectroscopy. In both cases, some experiments required temperatures down to -110°C. Cooling the sample slowed down an otherwise extremely fast rotation, facilitating the study of the different steps of the cycle in detail. ‘The design is clever [because] it incorporates a fluorine atom into the structure,’ explains Bismillah. This ‘tag’ added the possibility of using fluorine NMR, which tends to showcase simpler signals.
‘Fluorine NMR spectroscopy studies were very helpful,’ explains van Beek. ‘We could differentiate and follow all eight possible isomers using this technique,’ she says. Additionally, they carried out DFT studies to characterise the conformations of all isomers, particularly the double metastable intermediate, which is key to communication in the coupled rotors. Despite all efforts, the exact explanation for the communication mechanism remained elusive. Yet, the study provides a ‘fundamental understanding’ of the characteristics of coupling in molecular motors, says Bismillah, creating a solid base ‘before moving onto more complex systems [with] several motors working together, such as systems in biology’.
Van Beek says the isoindigo system is a small scale, molecular equivalent of bigger biological machinery like the ATP synthase enzyme, which also exhibits mechanically coupled motion. ‘Although the ATP synthase is clearly capable of much more sophisticated functions than our synthetic motor … the development and control of molecular-level motions will open many possibilities,’ she says. Maybe, artificial molecular motors could present properties ‘beyond biological and macroscopic machines … achieving new functionalities’.
References
CLF van Beek and B Feringa, J. Am. Chem. Soc., 2024, DOI: 10.1021/jacs.3c14430
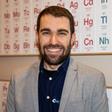
No comments yet