Fullerenes can catalyse the formation of hydrogen molecules in interstellar clouds. The finding could help to explain why space is brimming with molecular hydrogen despite cold environments that should make its formation improbable.
‘The formation of molecular hydrogen under astronomical conditions is the most fundamental chemical processes in the … universe, and the basis for evolution of more complex chemistry,’ explains molecular physicist Nigel Mason at the University of Kent, UK, who was not involved in the study. ‘However, it is also one of the most difficult to resolve.’
This is because the probability of two hydrogen atoms finding each other in the vast, cold vacuum of space is extremely low. Even if they did collide with enough energy to form a molecular bond, the reaction would release energy that the molecule would quickly re-absorb, causing the hydrogen to dissociate.
‘The formation of molecular hydrogen through direct collisions between two hydrogen atoms is negligible,’ says David McKenzie, a materials physicist at the University of Sydney in Australia. This means that the abundance of molecular hydrogen must be forming some other way.
Now, McKenzie and his colleague Yuzhen Guo think they’ve identified a key ingredient that could be helping hydrogen atoms to combine: fullerenes. These are spheroidal molecules made of 60 carbon atoms, shaped like tiny footballs.
It is widely accepted that dust grains could be acting as catalysts, providing a surface for hydrogen to absorb and bond more easily than they would when floating freely in space. Guo says they selected fullerene as their model surface because they have features in common with much of the carbon that makes up interstellar dust. ‘There is also a substantial amount of evidence supporting the existence of C60 molecules in space,’ he adds.
Using computational modeling, Guo and McKenzie explored two mechanisms for hydrogen formation on fullerene. In the first, two hydrogen atoms weakly attached to the surface, move around and eventually collide. In the second mechanism, an incoming hydrogen atom strikes another that is already chemically bonded to the surface, forming H₂ directly.
‘We also [found] that these surfaces do not encourage the reverse reaction, where molecular hydrogen is broken back down into single atoms,’ said McKenzie. Instead, the energy released during bond formation is absorbed by the fullerene, and ‘molecular hydrogen is repelled, preventing it from interacting with the surface’.
This is the first time this has been shown, comments Mason, who adds that it ‘is important in defining the chemical balance in H2 reactions’.
Guo and McKenzie’s modeling also showed that hydrogen formation through the mechanism involving chemical absorption of atomic hydrogen can occur at temperatures as low as 10K, filling a long-standing gap in temperature where neither mechanism (physical or chemical absorption) was previously thought to work. They also showed that these surfaces can capture high-energy hydrogen atoms, and that molecular hydrogen can still form efficiently at temperatures up to 6000K.
‘This offers insights into how molecular hydrogen is formed under energetic events in space,’ says Guo, like supernova shocks or under intense radiation from young stars, where hydrogen atoms can reach very high energies.
Mason calls the study an interesting contribution to a complex and still not fully understood astrochemical process. However, he points out that in many regions of space, dust grains are coated in ice, so modeling the reaction on icy surfaces would make the findings more realistic – though Guo and McKenzie argue that chemical absorption of hydrogen may occur before ice fully forms.
Mason adds that comparing the results with observable spectral data would help to substantiate the model. ‘Simulations alone are not sufficient to unravel the process,’ he adds. ‘To validate the production mechanism, experiments are necessary.’
References
Y Guo and D R McKenzie, Commun. Chem., 2025, DOI: 10.1038/s42004-025-01489-z
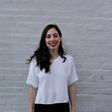
No comments yet