Next-generation lithium batteries with metallic lithium anodes and solid ceramic electrolytes may be one step closer after a study showed dendrites growing from the lithium anode and fracturing the ceramic electrolyte in a two-stage process. The work gives researchers useful leads on how to inhibit them and lengthen battery life.
Commercial lithium batteries today use an organic liquid electrolyte with a graphite anode. Lithium-ions are reduced and intercalate into the graphite structure when the battery is charged, and are oxidised and released on discharge. Charles Monroe, from the University of Oxford in the UK, describes the development of the intercalation anode as one of the ‘transformative discoveries’ in the development of the modern lithium battery. ‘Metallic lithium is terribly unstable – when you deposit it out of a liquid it looks like moss rather than a planar metal, and it’s also highly reactive, undergoing side reactions and capacity loss,’ he explains. However, intercalation anodes also have much lower capacity than pure lithium anodes.
Replacing the liquid organic solvent with an ionically conductive ceramic solid could potentially solve both problems: the ceramic should maintain the shape of the interface with a metallic lithium electrode and should reduce side reactions. However, researchers have found that tendrils of lithium called dendrites grow from the anode towards the cathode through the dense ceramic electrolytes. These also form in liquid electrolytes because cations are attracted to the closest electron source. Their occurrence in a ceramic, however, is puzzling. Monroe describes the texture of lithium as ‘more like cold butter than steel’. ‘Conventional thinking is that a soft material isn’t going to penetrate a hard material,’ says Monroe’s Oxford colleague Peter Bruce: ‘That’s the exam question: how is that possible?’ Such dendrites can fracture the material, leading to short circuit.
Previous research had suggested that lithium deposition into tiny cracks and pores in the ceramic could lead to dendrite formation. In the new work, however, a team surrounding Monroe and Bruce re-examined the process by incorporating the common argyrodyte electrolyte lithium phosphorus sulfur chloride into test batteries and following crack formation and propagation in real time using in operando x-ray computer tomography and multiple other imaging techniques. Using this data and extensive modelling, the researchers showed that fractures occurred in two stages, with cracks initially occurring at pores. However, once cracks were established, they propagated by being pulled apart at the base rather than sliced at the tip.
This could provide some useful hints for developing more durable solid battery electrolytes: ‘The mechanical properties that determine initiation are local fracture strength at the grain boundaries, whereas the mechanical property than determines propagation is the fracture toughness,’ explains Bruce. In particular, the researchers found that batteries operated under high pressure short circuited five times sooner: ‘It’s often thought that applying stack pressure to the cell would be a good thing, and the only problem with that is that you’d need more metal and more weight,’ he says; ‘What we’ve shown here is that, even if you could live with that overhead, you don’t want it.’
Materials scientist Kelsey Hatzell of Princeton University in the US says that the work ‘starts to – pun intended – chip away at this [dendrite formation] mechanism that’s pretty much not well understood.’ She says that the argyrodytes are just one class of ceramic electrolytes, albeit a very common one: ‘There are two next steps I would say: first is to understand whether this is universal to all solid electrolytes – which I don’t think it would be – and the second is to understand how this mechanism changes when you have a real intercalation cathode.’
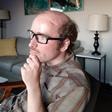
No comments yet