Bacteria are known to use ‘jumping genes’ to reorder their genome and fast-forward evolution. Now, scientists have discovered a mobile genetic element from this microbial world that might be able to rearrange large sections of our own DNA.1
This ‘takes us beyond the DNA and RNA cutting abilities of Crispr and RNA interference and towards a broader suite of capabilities’ for genome design, Patrick Hsu, geneticist at the University of California, Berkeley, said at a media briefing.
His group has found an enzyme that uses an RNA bridge in two parts: one binds to a sequence of donor DNA and another to the target DNA to insert the donor sequences. The discovery stemmed from investigations of a transposable element that can cut and paste itself into microbial genomes. The non-coding RNAs flanking the sequence controls an enzyme called a DNA recombinase.
‘This new study has dipped into bacterial genomes again and found some distant cousins of the Crispr–Cas genes known as recombinases,’ says Antony Adamson, who leads the Genome Editing Unit at the University of Manchester, UK. ‘Recombinases have been known for some time and can facilitate the rearrangement of very long DNA sequences, but to date none of these systems have been “programmable”.’
Recombinases are widespread in bacteria and this research focused on one found in some strains of Escherichia coli.
By engineering the target and donor RNA, the researchers were able to remove, insert and flip bacterial DNA sequences using this recombinase. ‘This programmable mechanism allows us to specify any two DNA sequences that we want to combine,’ says Hsu, delivering ‘an unprecedented level of controllability for manipulating genomes’.
Crispr was discovered in 1987 and developed as a gene-editing tool in 2012. Crispr with the Cas9 protein is akin to a molecular scissors that recognises and cuts a single target site. Crispr cuts DNA and then relies on repair machinery from the host cell to repair the sliced genome, which can result in long deletions or unexpected rearrangements. The new RNA-bridging mechanism completes its DNA edit in a single step. Another advantage is that the recombinase protein is smaller than many Cas enzymes of the Crispr system, making it easier to pack editing machinery into the viruses needed to deliver into human cells.
A big drawback of Crispr is our inability to control repair, says Adamson. ‘This means we may have to screen many extra cells to find ones with the desired outcome,’ he notes. ‘If someone wanted to treat a person with a genetic condition then the lack of precision presents a huge safety issue.’
In a second paper, the US team collaborated with structural biologist Hiroshi Nishimasu at the University of Tokyo, Japan.2 They revealed that the cryo-electron microscope structures of the recombinase complexed with its bridge-DNA, target DNA and donor DNA at different stages of the recombination.
Separately, a team in Sydney reported on the structure of the recombinase and a family of related molecules, also highly selective and programmable, which they term SeekRNA.
The new bridge-DNA mechanism has only been demonstrated in bacteria so far. The next challenge is to put it to work in mammalian cells. ‘If this is successful, then very precise, large-scale alterations of mammalian cells may become possible,’ says Adamson.
‘This is an important step forward towards the broader vision of genome design, where we’ll one day want to change not just individual or hundreds of bases, but thousands, tens of thousands, hundreds of thousands, millions of bases,’ Hsu explains.
References
1 MG Durrant et al, Nature, 2024, 630, 984, (DOI: 10.1038/s41586-024-07552-4)
2 M Hiraizumi et al, Nature, 2024, 630, (DOI: 10.1038/s41586-024-07570-2)
3 R Siddiquee et al, Nat. Commun., 2024, 15, 5235, (DOI: 10.10 38/s41467-024-49474-9)
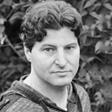
No comments yet