An investigation into a neutron-rich isotope of indium using a cutting-edge nuclear physics technique has begun to unravel the mysteries of how single particles behave inside the nucleus.
We have known that a nucleus is comprised of protons, which give an element its atomic number, and neutrons since the early 1930s. But how an individual proton or neutron behaves inside the heart of an atom is still poorly understood. Now, an international collaboration including scientists from Canada, China, Finland, France, Germany, Poland, Sweden, Switzerland, the UK and US has taken a step closer to understanding these complex interactions.
Nuclear physics researchers often look at elements with so-called ‘magic numbers’ of protons or neutrons, which are exceptionally well bound and thus highly stable. However, to learn about nuclear structure, nuclides with one fewer proton are used, known as a single proton hole. By investigating the electronic transitions, researchers can study the atomic, hyperfine structure of individual particles due to the interactions between electrons and the nucleus. This gives clues as to the nucleus’ magnetic and electric characteristics, which can then give a complete picture of how all protons and neutrons are distributed and interact inside a nucleus.
To do this, the researchers looked at indium-131, which has a magic number of neutrons (82) and a single proton hole (tin, with 50 protons, is also magic). However, as indium-131 has 16 neutrons more than its most common isotope, it’s incredibly unstable – only lasting around 0.28 seconds. The team therefore had to use a cutting-edge technique called collinear resonance ionisation spectroscopy, performed at the Isotope Mass Separator On-Line Facility (Isolde) at Cern in Switzerland, to obtain experimental results.
Klaus Blaum calls the work ‘wonderful’. Blaum wasn’t involved in this work but is director of the Max Planck Institute for Nuclear Physics in Germany. ‘The detection sensitivity has been pushed such that less than 1000 atoms per second are sufficient to perform the experiment. This allows us to probe even very exotic radioactive short-lived species, like indium-131 reported here,’ he says.
These experiments, which were confirmed by two different approaches to theoretical modelling, demonstrate an effective way for researchers to map how the nucleus is held together and how each of its component particles interacts with the others.
‘The results … provide a deep insight into the interaction of a single particle (or a single hole) with the collective behaviour of the other [protons and neutrons], thus improving our understanding of complex many-body systems significantly,’ adds Blaum.
Following the indium-131 experiments, the researchers intend to continue their investigation into short-lived isotopes to better understand how nucleuses are held together, and the complex forces at play in the heart of every atom.
References
A R Vernon et al, Nature, 2022, DOI: 10.1038/s41586-022-04818-7
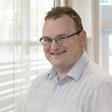
No comments yet