Scientists are turning to supramolecular chemistry to design new, more efficient and flexible molecular qubits for quantum technologies, like quantum computing.
‘A qubit is the fundamental unit of quantum information,’ explains Sabine Richert at the University of Freiburg. Unlike classical computers, which use a magnetic material to store and process information as 1s or 0s, quantum computers rely on qubits that can exist in a superposition of two or more quantum states at once. This enables quantum computers to process many possibilities simultaneously, making them better at solving certain problems that would take classical computers years.
While many quantum computers use superconductors, trapped ions or photons to create qubits, molecular qubits offer an interesting alternative. By exploiting molecular properties, like electronic and nuclear spin states, these systems can store and manipulate quantum information. Advantages include tunability through molecular design and longer coherence times, which allows qubits to maintain their quantum state – and the information they hold – for longer.
So far, scientists have primarily explored molecules with strong, rigid chemical bonds for this purpose. ‘Before [our study], it was widely accepted that a covalent bond was necessary for a strong interaction between two units with unpaired electrons,’ says Andreas Vargas Jentzsch at the University of Strasbourg.
However, Richert, Vargas Jentzsch and their colleagues wanted to know if weaker, reversible interactions – specifically hydrogen bonding – could offer a more flexible way to control the interaction between quantum spins.
To create their hydrogen-bonded molecular qubits, the team used a chromophore–radical system, the magnetic properties of which make it a good candidate for quantum manipulation – even at room temperature. ‘Chromophore–radical systems are photogenerated model qubits, also called qu-d-its, as they can have more than two spin states,’ explains Vargas Jentzsch.
Molecular qubits, or qudits, are unique because they can hold multiple qubits within a single molecule, creating a larger space for storing and processing information, compared with conventional qubits.
‘Quantum error correction operations, which are the bottleneck of current quantum computers and the reason why their impact on the market is still quite limited, are much more efficient in qudits,’ says Lorenzo Tesi at the University of Stuttgart, who was not involved in the study.‘[Ours is composed of] a chromophore, which is a colourful dye, and a stable radical,’ says Vargas Jentzsch. ‘The radical possesses an unpaired electron, representing a doublet spin state … and when we promote the chromophore to its excited state, we generate a triplet excited state with two unpaired electrons.’
The resulting triplet–doublet system interacts through the hydrogen bond molecular bridge, through which the system’s spins become entangled and ‘mix’ – referred to as spin mixing – to yield a ‘quartet state’ with four possible configurations that can be individually controlled using microwave radiation.
‘This methodology is one of the most promising in the field,’ Tesi says. ‘The advantages … include high spin polarisation, which makes it possible to work at temperatures hundreds of times higher than those possible with superconducting qubits, high sensitivity … and the ability to address the qubits spatially.’
The chromophore–radical systems were self-assembled using supramolecular chemistry, a process that relies on non-covalent interactions to bring molecules together. This approach not only allows precise control over the structure and properties of the system but has the added advantage of scalability to create larger, more complex systems.
The team also found that the spin mixing in the hydrogen-bound molecules was even stronger than an equivalent, covalently-bound system with a comparable separation between the same chromophore–radical building blocks. ‘The properties [were] comparable to, or even superior than, those of their fully covalent counterparts,’ says Richert. ‘The only way this is possible is if the hydrogen bonds truly serve as effective bridges for the transmission of quantum (spin) information.’
Tesi believes this is an important step in showing that non-covalent interactions play a key role in coupling spin systems, which could expand the range of possible molecular qubits.
However, we are still in the early stages of exploring these new possibilities. Richert and Vargas Jentzsch believe that practical applications are still a long way off. ‘While quantum computing is the best-known application of qubits, it is not the only field in which qubits can be used,’ says Richert. ‘It would almost be fair to say that it is one of the applications that is still not within reach. One of the applications that appears most realistic is quantum sensing.’
‘Ultimately, the flexibility of molecular spin qubits gives us unlimited possibilities to design new structures and the means to control them,’ she adds.
References
IV Khariushin et al, Nat. Chem., 2025, DOI: 10.1038/s41557-024-01716-5
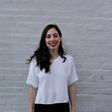
No comments yet