At the Port of Los Angeles in California, two barges filled with tanks and pipework provide an unlikely setting to open up a new frontier to tackle the climate crisis. The start-ups running them are part of a growing effort to harness the power of the ocean to capture carbon dioxide from the air.
The ocean is a massive carbon sink, having absorbed around 30% of the carbon dioxide we’ve pumped into the atmosphere. Marine life is already paying a terrible price for the resulting changes in ocean chemistry though. Carbon dioxide reacts with seawater forming carbonic acid, which in turn dissociates into bicarbonate and hydrogen ions. The more dissolved carbon dioxide, the more positively charged hydrogen ions. This results in increased acidity that in turn can dissolve the shells and skeletons of sea creatures.
Both Captura, a spin-out from the California Institute of Technology, and Equatic, from the University of California, Los Angeles, have bold plans to address climate change by removing carbon dioxide from the world’s oceans and sequestering it. They are confident that their electrochemical systems will eventually remove carbon dioxide for less than $100 (£80) a tonne. The US Department of Energy is targeting $100 a tonne for carbon removals by 2032. How quickly that milestone can be reached will depend on how fast the technology can scale and the future costs of renewable energy, but Equatic anticipates it can achieve this by 2028. For comparison, Climeworks, which has a 4000 tonne per year direct air capture plant running in Iceland, expects it can get costs down to around $250–$300 a tonne by the end of the decade.
Ocean capture technologies are already attracting significant investment. Over the past year Captura has raised more than $33 million to commercialise its technology. Investors include Norway’s state-owned energy giant Equinor, with whom it’s developing a 1000 tonne plant on Norway’s west coast. The plant will test the full end-to-end operation, and the carbon dioxide captured injected under the seabed for permanent storage. Captura’s first commercial plant is slated to remove 50,000 tonnes of carbon dioxide per year.
Both technologies are closed systems, so the amount of carbon dioxide captured can be precisely measured. This is critical to achieving large scale carbon removal. An industry is already developing, with companies purchasing removals in advance. Boeing, for example, has signed a five-year agreement with Equatic to purchase 62,000 tonnes of carbon removals and 2100 tonnes of green hydrogen that will be produced by the process. Some estimates suggest the deal is worth at least $50 million.
Cutting energy consumption
By volume, seawater holds 150 times more carbon dioxide than the air, making it potentially more efficient to remove it from the sea. But water is heavier. ‘It costs more to move, but it doesn’t cost 150 times more,’ says Steve Oldham, chief executive of Captura.
But the energy costs of pumping seawater are considerable. Pumping each cubic metre of water into a desalination plant can require around 0.45kWh of electricity. Based on the composition of seawater, Equatic estimates that it can pull 4.6kg of carbon dioxide from every cubic metre of water. That would imply a plant capturing 1 million tonnes would require 97GWh of renewable energy, just to pump seawater. In future, there may be cheaper ways to move water, such as harnessing tides or ocean currents, but for now the process remains energy intensive and costly. Co-locating a carbon capture plant alongside a desalination plant would cut energy requirements for both plants, but only by 9%, according to Equatic’s laboratory studies.
Both Captura and Equatic rely on electrochemical processes to extract carbon dioxide. And that’s where the bulk of their energy costs fall.
Equatic’s system captures carbon dioxide in the form of carbonate minerals and produces clean hydrogen through the electrolysis of seawater. When it comes to carbonate formation Gaurav Sant, director of the Institute for Carbon Management at UCLA and a co-founder of Equatic, says that ‘we’re just mimicking what the oceans do’.
Electrolysis eats up 80–90% of Equatic’s total energy needs. ‘Even though you’re moving a really large quantity of water, electrolysis is so much more energy intensive. That’s basically the elephant in the room,’ he adds.
From an initial lab system, they’ve reduced the overall energy requirement of the process by over 10% to around 2MWh/tonne of carbon dioxide captured. Some 10 gigatonnes of emissions may need to be removed to stay within the Paris Agreement limits, implying 20,000TWh of renewable electricity would be required by 2050 for removals by this route alone. The US generated 974TWh of renewable electricity in 2022.
On the other side of the equation, Equatic’s process also produces hydrogen. Standalone generation by today’s electrolysers require between 42–65kWh/kg of hydrogen, and a supply of fresh water. However, energy will be required to compress and transport the hydrogen produced, unless it’s used in a fuel cell to power the system.
The electrodes Equatic has developed take the form of sheets for maximum surface contact with seawater and are made of common alloys and small amounts of platinum group metals. Normally, electrolysis of seawater would lead to the production of chlorine, but the researchers can now suppress its formation. ‘This is a really major breakthrough,’ says Sant. ‘We’ve spent a lot of time and effort on electrode engineering and electrode design to allow the Equatic process to be as energy efficient as possible, but also to open up the entire pathway to direct seawater electrolysis for hydrogen production.’
Carbonate chemistry
To capture carbon dioxide, the system takes advantage of the abundance of magnesium and calcium ions in seawater. These bind carbon dioxide at the cathode to produce calcium carbonate, and hydrated magnesium carbonates – so trapping carbon dioxide that was present in seawater. These can be continuously ‘scraped’ off the electrode mesh. Air is then bubbled through the resulting alkaline, carbon dioxide-depleted stream of seawater so that more carbon dioxide is removed from the atmosphere.
At the anode, the acid stream of seawater must have its alkalinity restored before it can be returned to the sea. This can be done by adding abundant minerals such as olivine. Coastal weathering deposits minerals in the ocean, helping to restore alkalinity, but oceans today are absorbing carbon dioxide at a faster rate than this natural process can compensate for. The addition of alkaline minerals is being pursued by other companies, but calculating the resulting carbon dioxide drawdown is fraught with uncertainty.
Simply returning the mineral carbonates to the ocean avoids any of the compression and transport costs of carbon sequestration. While Equatic may ‘just’ be speeding up a natural process, no one knows just how much it can be accelerated without an adverse impact on ecosystems. It’s estimated that the deep ocean already stores some 37,000Gt of carbon. ‘Our position is to carry out really detailed environmental impact assessments, to be able to study exactly what is happening in exquisite detail and gain more information as [we] go along,’ says Sant.
Alternatively, the carbonate minerals could be used in construction or to shore up coastlines affected by rising sea levels, although energy would be required to transport them from the plant.
Softening up carbon capture
In Captura’s process, filtered seawater is constantly moving through the plant. Crucially, to reduce energy consumption, only 0.5% of the seawater intake goes through the electrodialysis step. It’s first softened (to remove magnesium and calcium ions), and then passed through a stack of electrified membranes. The materials are under wraps for now, but Oldham says they contain no rare earth elements.
The water molecules dissociate into hydroxyl and hydrogen ions. The acid stream is added back into the main flow of seawater going through the plant, where it goes from pH8.1 to around 4. The change in acidity causes bicarbonate ions to give up carbon dioxide, which is removed from the water via a gas–liquid membrane contactor and a vacuum pump. Tests suggest capture rates are around 95%. To further improve the efficiency of the process Captura is working with industry to develop a membrane that doesn’t remove oxygen from the water.
The other (alkaline) stream emerging from the electrodialysis process is then added back into the water to neutralise the acidity and bring the pH back up to around 8.1. Because carbon dioxide has been removed from the water coming out of the plant it should draw down more from the atmosphere – provided it remains at the ocean surface. Exactly how much will be absorbed by the surface waters is yet to be definitively proven.
However, the electrodialysis step is very energy intensive – absorbing around 70% of total process energy requirements. Captura has not published figures but to reduce energy demand and cut costs, it plans to over-build the electrodialysis unit so that it can run on off-peak renewables. Using off-peak electricity for six hours a day, enough acid and base could be generated to keep the process going for 24 hours. Another bonus is that it should quadruple the lifetime of the membranes. Today’s commercially available membranes have a lifetime of over 10,000 hours.
‘We’re pretty close to the thermodynamic limit in the performance of electrodialysis,’ says Oldham, but ‘we will continue to work on the physical size of the membrane – the bigger, the better, but you have to maintain performance’. An additional energy cost will be incurred to compress and transport carbon dioxide for storage. One lifecycle analysis put the energy for compression at 111kWh/tonne of carbon dioxide captured, and the energy to inject it into a well at 7kWh/tonne.
A recent analysis suggests pairing these processes with desalination would limit the carbon dioxide removal potential to less than 10 million tonnes a year. However, such plants are in regions like the Middle East, with the potential to scale the solar energy to power them.
For Captura co-locating is a ‘get to market strategy’. After that, it would have to scale the technology independently. It hopes its new partnership with Equinor will enable it to tap into the state petrochemical firm’s experience of building structures such as rigs out at sea.
While the industry focuses on scaling and demonstrating that its systems do benefit ocean health, the issue of cost remains. ‘There’s no question about why it’s important. There’s no question it needs to be done,’ says Sant. ‘The question is, how are we going to pay for it? And who is going to pay for it?’
References
IA Digdaya et al, Nat. Commun., 2020, 11, 4412 (DOI: 10.1038/s41467-020-18232-y)
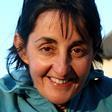
No comments yet