Exquisite insight into chromosome separation reveals the intricate relationships between molecular changes and large-scale cell processes
We should never underestimate the ingenuity with which biology uses molecules to get stuff done. When the Nobel laureate François Jacob wrote in 1970 that ‘the aim of modern biology is to interpret the properties of the organism by the structure of its constituent molecules’, he was articulating the programme that had existed ever since it became clear in the 1950s how those structures could be encoded in the genome sequence. What this amounted to was privileging a certain length scale in the biological hierarchy: that of the molecule and its spatial arrangement of atoms. In this view, everything followed from that structure.
Today a more pluralistic view is emerging in which no length scale is privileged. That’s to say, the organisation on which life depends operates at all sizes from the atomic to the organismal, with a distinct set of principles at each level of magnification. This organisation is not truly built from the bottom up, all encoded in the genome sequence, but rather that molecular structure typically fine-tunes outcomes already inherent in the meso- and macroscale properties of matter. Genes exemplify this notion: they don’t exactly dictate morphology but rather determine why one morphology differs from another.
Among very many illustrations of this picture, that recently reported by a team at the European Molecular Biology Laboratory in Heidelberg, Germany, led by Sara Cuylen-Haering, is particularly wonderful and enlightening.1 The researchers have investigated how chromosomes are sequestered during cell division. We know the usual story: the chromosomes, condensed into their compact X-shaped structures, are arranged on the microtubule filaments of the mitotic spindle, where they are apportioned half into each daughter cell. But there’s more to it. To begin with, each chromosome must be kept separate from the others. Yet in the late stage of division (anaphase) the spindle begins to disappear and the chromosomes clump together for bundling up into the new nuclei, without being caught up with any detritus in the surrounding cytoplasm.
This is a process operating on a scale of a few micrometres, and so it demands forces beyond the scope of individual molecules. The cell seems to use colloidal phenomena such as surface tension, known to be mediated by a protein called Ki-67 that has long been used as a marker of cell proliferation. Perplexingly, Ki-67 seems essential both to keep chromosomes apart earlier in mitosis and to their clustering in the late stage: it seems to switch its function during cell division.
Using live-cell imaging, in vitro experiments and simulations, Cuylen-Haering and colleagues reveal what seems to be going on. It’s a splendid example of how mesoscale processes in biology are tuned and switched at the molecular scale. At the start of mitosis, Ki-67 is highly phosphorylated – the protein is dotted with phosphoryl groups that give it segments with alternating charge that is negative overall. These molecules form a brush-like layer on the surface of the chromosomes, pushing them apart much as polymer surfactants are used in lubrication.2
But during the exit stage of cell division, enzymes dephosphorylate Ki-67, rendering it positively charged. This change enables the protein to bind with RNA molecules (which are polyanions) in the nucleus and phase-separate into dense, liquid-like droplets that can spread over the chromosome surfaces. Fusion of this liquid layer on different strands then pulls them together, much as wet hair clumps together.
The protein-RNA clusters are basically biomolecular condensates, now known to be ubiquitous in cell biology3 albeit here gathering as a sort of wetting film on the chromosomes that binds them via surface tension. Many condensates are formed from proteins and ‘noncoding’ RNAs – that is, ones that don’t encode proteins as messenger RNA. But the RNA in this case is the first noncoding RNA to be recognised as such: ribosomal RNA (rRNA), which generally constitutes part of the ribosome complex responsible for translating mRNA into protein. Here rRNA serves a quite different role, and does so while still ‘premature’, before enzymes snip it up and prepare it for duty in the ribosome.
Frankly, one glance at Ki-67 will, to the practised eye, suggest something like this might be going on. For the protein has all the hallmark features of those that tend to be involved in phase separation and condensate formation: it has a lot of structural disorder and is multivalent, containing several ‘sticky patches’. Phosphorylation and other such modifications are a common mechanism by which these relatively loosely folded proteins can be shape-shifted and tuned to alter their propensities for other molecules. We now know that evolution has ‘designed’ many proteins like these for collective condensation – and that, contra Jacob, that’s not so much about molecular-scale structure as lack of it. One might say that such molecules function not as tiny machines but rather as components of material systems that allow cells to control their mesoscale organisation.
References
1 A Hernandez-Armendariz et al., Mol. Cell, 2024, 84, 3254 (DOI: 10.1016/j.molcel.2024.07.022)
2 T Kreer, Soft Matter, 2016, 12, 3479 (DOI: 10.1039/C5SM02919H)
3 S F Banani et al., Nat. Rev. Mol. Cell Biol., 2017, 18, 285 (DOI: 10.1038/nrm.2017.7)
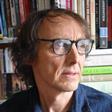
No comments yet