The global push towards net zero emissions has gained unprecedented momentum in recent years, driven by the urgent need to address climate change and its far-reaching consequences. As nations, industries and organisations worldwide commit to ambitious carbon reduction targets, chemistry has a critical role to play in achieving these goals.
The net zero landscape
The Paris Agreement, adopted in 2015, set the stage for global action on climate change, aiming to limit global warming to well below 2°C, preferably 1.5°C, compared to pre-industrial levels. As of 2023, over 70 countries, including major economies like the US, China and the EU, have set net zero targets, many pledging to achieved net zero emissions by the middle of this century .
In parallel with governmental action, the private sector has seen a surge in net zero commitments. As of 2023, over 3000 businesses have joined the UN’s Race to Zero campaign, pledging to achieve net zero emissions by 2050 or earlier. This includes many Fortune 500 companies and industry leaders across sectors such as energy, manufacturing, technology, and finance.
These corporate pledges are driving significant changes in business strategies, investment patterns, and technology development. They are also creating new markets for low-carbon solutions and sustainable technologies, many of which rely heavily on chemical innovations.
Despite the widespread adoption of net zero targets, challenges and criticisms remain. These include concerns about the lack of standardised methodologies for measuring and reporting emissions, the reliance on future technologies for carbon removal and the potential for ‘greenwashing’. There is also ongoing debate about the equitable distribution of the burden of emissions reductions between developed and developing countries, and between different industry sectors. These challenges underscore the need for robust scientific approaches, including those from the chemical sciences, to provide verifiable and effective solutions.
The role of chemistry in net zero
Chemistry plays a crucial role in reaching the goal of net zero, particularly in technologies and sectors such as renewable energy, carbon capture, materials, agriculture and transport.
Renewable energy the development and improvement of renewable energy technologies, which are fundamental to achieving net zero goals.
Solar energy
Recent trends in solar energy focus on improving the efficiency and reducing the cost of photovoltaic (PV) cells. Chemical research is at the forefront of these efforts.
Perovskite solar cells: these emerging PV materials have seen rapid improvements in efficiency, reaching over 25% in laboratory settings. Current research focuses on enhancing their stability and scaling up production. Chemical innovations in materials design and synthesis are key to overcoming these challenges.
Tandem solar cells: combining different PV materials to capture a broader spectrum of light is a promising approach to boost efficiency. For instance, perovskite-silicon tandem cells have achieved efficiencies over 29%. Chemistry is crucial in developing compatible materials and interfaces for these multi-junction cells.
Organic photovoltaics: while less efficient than silicon-based cells, organic PV materials offer advantages in flexibility and low-cost production. Recent chemical research has focused on developing new organic semiconductors and optimizing their molecular structures for improved performance.
Energy storage
Efficient energy storage is critical for managing the intermittency of renewable sources. Battery technology is therefore a key focus area.
Lithium-ion batteries: continuous improvements in electrode materials and electrolytes have led to higher energy densities and longer lifespans. Recent trends include the development of silicon anodes and solid-state electrolytes.
Flow batteries: these large-scale storage systems are gaining attention for grid-level applications. Chemical research is focused on developing more efficient and cost-effective electrolyte materials.
Hydrogen storage: as interest in hydrogen as an energy carrier grows, chemistry is central to developing safe and efficient storage methods, including metal hydrides and chemical hydrogen carriers.
Wind energy
While primarily a mechanical technology, wind energy also benefits from chemical innovations.
Advanced composites: development of stronger, lighter materials for turbine blades, including carbon fibre reinforced polymers and novel resins, is an active area of chemical research.
Corrosion-resistant coatings: for offshore wind installations, specialised coatings to protect against saltwater corrosion are crucial.
Carbon capture, utilisation and storage (CCUS)
CCUS technologies are increasingly seen as essential for achieving net zero, particularly for hard-to-abate sectors. Chemistry is at the heart of CCUS innovation.
Carbon capture
Recent trends focus on improving the efficiency and reducing the cost of capture technologies.
Advanced sorbents: development of novel materials with high CO2 selectivity and capacity, such as metal-organic frameworks (MOFs) and amine-functionalized porous materials.
Membrane separation: research into high-performance polymeric and mixed-matrix membranes for selective CO2 separation from flue gases or ambient air.
Direct air capture (DAC): Chemical innovations in sorbent materials and regeneration processes are key to making DAC more energy-efficient and cost-effective.
Carbon utilisation
Converting captured CO2 into valuable products is an area of growing interest.
Carbon dioxide to fuels: catalytic processes for converting CO2 to methanol, syngas or other hydrocarbon fuels are being developed, with a focus on improving catalyst efficiency and selectivity.
Carbon dioxide to chemicals: synthesis of high-value chemicals like polymers, carbonates, and carboxylic acids from CO2 is an active research area.
Mineralisation: processes for converting CO2 into stable mineral carbonates for use in construction materials are being scaled up.
Carbon storage
Safe and permanent storage of captured CO2 is crucial to mitigate the climate changing effects of global warming.
Geological storage: chemical research focuses on understanding and optimizing CO2 -rock interactions, developing monitoring techniques, and ensuring long-term stability of stored CO2.
Enhanced oil recovery (EOR): While controversial due to its role in fossil fuel extraction, CO2 -EOR is being explored as a way to offset capture costs while permanently storing CO2.
Sustainable materials and the circular economy
The transition to a circular economy is a key component of many net zero strategies, with chemistry playing a central role in materials innovation and recycling technologies.
Bio-based and biodegradable materials
Bioplastics: development of new polymers from renewable feedstocks, such as PLA (polylactic acid) and PHA (polyhydroxyalkanoates), with improved properties and biodegradability.
Cellulose-based materials: innovations in processing technologies to create advanced materials from cellulose, including nanocellulose for high-performance applications.
Bio-based building materials: development of low-carbon alternatives to cement and concrete, such as bio-based binders and aggregates.
Advanced recycling technologies
Chemical recycling: processes like depolymerization and solvolysis are being developed to break down plastics into their chemical building blocks, enabling true circular recycling.
Upcycling: chemical processes to convert waste materials into higher-value products, such as turning plastic waste into carbon nanotubes or graphene.
Additive separation: development of chemical techniques for efficient separation of complex material mixtures, enabling better recovery of valuable components from waste streams.
Sustainable synthesis and manufacturing
Green chemistry principles: concepts such as atom economy, waste reduction and safer solvents being adopted in industrial processes.
Biocatalysis: use of enzymes and microorganisms for more efficient and environmentally friendly chemical synthesis.
Continuous flow chemistry: development of more efficient and scalable chemical processes with reduced energy and solvent use.
Sustainable agriculture and food production
Chemistry plays a crucial role in developing sustainable agricultural practices and alternative food production methods, which are essential for reducing emissions from the agriculture sector.
Precision agriculture
Smart fertilisers: development of controlled-release fertilisers and nanofertilisers for more efficient nutrient delivery and reduced runoff.
Soil health: chemical analysis and enhancement of soil microbiomes for improved crop yields and carbon sequestration.
Pest management: design of more targeted and environmentally friendly pesticides and biopesticides.
Alternative proteins
Plant-based proteins: chemical innovations in protein extraction, texturisation, and flavour development for meat alternatives.
Cultured meat: development of growth media and scaffolds for cell-based meat production.
Microbial proteins: optimisation of fermentation processes for production of single-cell proteins from microalgae, fungi, or bacteria.
Food waste reduction
Active packaging: development of smart packaging materials that extend shelf life and indicate freshness.
Edible coatings: creation of biodegradable coatings to protect fruits and vegetables from spoilage.
Valorisation of food waste: chemical processes to extract valuable compounds from food waste streams.
Sustainable Transportation
The transportation sector is a major contributor to global emissions, and chemistry is key to developing low-carbon alternatives.
Electric vehicles (EVs)
Battery technology: ongoing research into new battery chemistries, including solid-state batteries, lithium–sulfur batteries and sodium-ion batteries for improved performance and sustainability.
Lightweight materials: advanced composites and high-strength alloys can reduce vehicle weight and increase efficiency.
Fuel cells: improvements in catalyst materials and membrane technology for hydrogen fuel cells.
Sustainable aviation fuels (SAF)
Biofuels: development of advanced biofuels from non-food biomass and waste streams.
Synthetic fuels: chemical processes such as Fischer–Tropsch for producing drop-in fuels from CO2 and renewable hydrogen (e-fuels).
Hydrogen aviation: research into safe storage and use of hydrogen in aircraft, including cryogenic technologies and fuel cell systems.
Maritime transportation
Alternative fuels: development of low-carbon fuels for shipping, including ammonia, methanol and biofuels.
Fuel cells: adapting fuel cell technologies for marine transport applications.
Carbon capture: on-board carbon capture systems for large vessels.
Emerging trends and future directions
In recent years, a number of new research areas and concepts have emerged that have the potential to transform chemistry and the sustainability of its products and processes.
Artificial intelligence (AI) and machine learning (ML) in chemistry
The integration of AI and ML techniques in chemistry research is accelerating the discovery and optimization of materials and processes for net zero applications.
Materials discovery: high-throughput computational screening of novel materials for energy storage, catalysis, and carbon capture.
Process optimisation: ML algorithms for optimizing complex chemical processes, reducing energy consumption and waste.
Predictive maintenance: AI-driven systems for predicting and preventing equipment failures in chemical plants, improving efficiency and safety.
Quantum chemistry and computational modelling
Advances in quantum computing and computational chemistry are enabling more accurate simulations of complex chemical systems.
Catalyst design: quantum chemical modelling for designing more efficient catalysts for CO2 reduction and hydrogen production.
Materials properties: accurate prediction of material properties for battery electrodes, solar cells, and gas separation membranes.
Reaction mechanisms: elucidation of complex reaction pathways in atmospheric chemistry and carbon cycle processes.
Synthetic biology and metabolic engineering
The convergence of chemistry and biology is opening new avenues for sustainable production of chemicals and materials.
Carbon fixation: engineering of microorganisms for efficient capture and conversion of CO2 into valuable products.
Biomanufacturing: development of cell-free systems and synthetic organisms for production of chemicals, fuels and materials.
Biomimetic materials: design of materials inspired by biological systems for enhanced sustainability and functionality.
Advanced characterisation techniques
New analytical tools are providing unprecedented insights into chemical processes and materials.
In-situ and -operando spectroscopy: real-time monitoring of chemical reactions and material transformations under realistic conditions.
High-resolution imaging: advanced microscopy techniques for visualising atomic-scale structures and processes in energy materials.
Big data analytics: integration of multi-modal characterisation data for comprehensive understanding of complex chemical systems.
Modular and distributed chemical manufacturing
Trends towards more flexible and localized production are changing the landscape of chemical manufacturing.
Microreactors and flow chemistry: development of compact, modular systems for on-demand production of chemicals and pharmaceuticals.
Additive manufacturing: 3D printing of chemical reactors and process equipment for rapid prototyping and customisation.
Decentralised production: distributed networks of small-scale chemical plants for reduced transportation and increased resilience.
Policy implications and recommendations
To achieve net zero goals, policies that foster and support research and innovation are also essential.
Research and development funding
- Increased public funding for basic and applied research in key areas such as CCUS, advanced materials and sustainable chemistry.
- Establishment of public–private partnerships to accelerate the commercialisation of promising technologies.
- Support for interdisciplinary research initiatives that combine chemistry with other fields like materials science, biology and computer science.
Regulatory frameworks
- Development of standardised methodologies for carbon accounting and life cycle assessment of chemical products and processes.
- Implementation of carbon pricing mechanisms to incentivise low-carbon innovations in the chemical industry.
- Harmonisation of international standards for sustainable and circular economy practices.
Education and workforce development
- Integration of sustainability principles and green chemistry concepts into chemistry curricula at all levels.
- Support for reskilling and upskilling programs to prepare the workforce for the transition to a low-carbon chemical industry.
- Promotion of diversity and inclusion in STEM fields to broaden the talent pool for sustainable chemistry innovation.
Infrastructure and supply chains
- Investment in infrastructure for renewable energy integration, CCUS deployment and hydrogen production and distribution.
- Development of secure and sustainable supply chains for critical raw materials needed for clean energy technologies.
- Support for the establishment of chemical recycling facilities and circular economy hubs.
International cooperation
- Facilitation of international research collaborations and knowledge sharing in sustainable chemistry.
- Support for technology transfer and capacity building in developing countries.
- Coordination of global efforts to address challenges in hard-to-abate sectors of the chemical industry.
Conclusion
The journey towards net zero emissions presents both challenges and opportunities for the chemical sciences. Chemistry is playing a pivotal role across a wide range of areas critical to achieving carbon neutrality, from renewable energy and carbon capture to sustainable materials and circular economy practices.
Recent trends show a rapid acceleration in the development and deployment of low-carbon technologies, driven by a combination of policy pressures, corporate commitments and technological breakthroughs. The chemical industry, with its unique position at the nexus of many value chains, has the potential to be a key enabler of the net zero transition across multiple sectors.
However, realising this potential will require sustained effort and collaboration among researchers, industry leaders and policymakers. It will necessitate not only technological innovations but also systemic changes in how we produce, use and recycle chemicals and materials.
For working chemists, these trends present exciting opportunities to contribute to solving one of the most pressing challenges of our time. Whether in academia, industry or government, chemists have a crucial role to play in developing the solutions needed for a sustainable, low-carbon future.
For industry, the net zero transition is a challenge to reinvent business models and value chains. Companies that can successfully navigate this transition, leveraging chemical innovations to develop sustainable products and processes, will be well-positioned for long-term success in a carbon-constrained world.
For policymakers, the complex landscape of net zero chemistry underscores the need for informed, science-based decision-making. Policies that support research and development, incentivise sustainable practices, and facilitate the deployment of low-carbon technologies will be crucial in accelerating the transition.
Chemistry will continue to be at the forefront of the net zero revolution. By harnessing the power of chemical innovation, we can create a more sustainable, resilient and prosperous future for all.
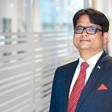
No comments yet