Identifying the PKZILLAs, used by algae to make toxins, stretched the capabilities of current analytical methods – and the limits of our preconceptions
For natural-product chemists, polyketides offer a high-stakes game. These typically polycyclic fused-ring carbon molecules are made by bacteria, algae, plants, fungi and some animals for a variety of purposes. Many polyketides are highly toxic, and they are key culprits in the devastating ecological effects of algal blooms that have episodically decimated local fish populations around the world. That toxicity, however, is partly what makes some polyketides candidate anticancer agents, which in turn makes them attractive targets for total synthesis.1 But one look at the complex structures, with their plethora of chiral centres, and the magnitude of that task is clear.
Never more so than for the prymnesins, which are some of the largest non-polymer organic molecules found in nature. The polyketide prymnesin-1 contains 90 carbon atoms, many arranged in a ‘ladder-frame’ of fused rings. It is made by the alga Prymnesin parvum, deadly blooms of which have occurred from Texas to Poland. It would be a brave or foolhardy chemist who even contemplates total synthesis – but it would surely be handy first to know how the molecule is assembled in nature.
Prymnesins are made by enzymes called polyketide synthases (PKSs). But researchers have struggled to identify the enzymes in question. Now Timothy Fallon at the Scripps Institution of Oceanography in La Jolla, California, and his coworkers have figured out why.2 This immense molecule is made by two gigantic enzymes, bigger even than the longest known protein titin that gives muscles their elasticity. The enzymes and their corresponding genes are so big that previous searches simply couldn’t recognise them as such. In recognition of their monstrous scale, the researchers call them PKZILLA-1 and PKZILLA-2.
Typically, PKSs work as assembly lines in which the molecule is put together in successive steps by modules each acting as a kind of enzyme in its own right. The PKZILLAs are like this, containing domains that transfer an amino acid or a methyl group, form a thioester, reduce a keto group, and so on. The nascent product molecule is essentially passed from one domain to another as a steadily growing and ever more complex chain. Doing the synthesis in this assembly-line way on a single giant enzyme enables precise control of the structure and stereochemistry of the product. (Additional enzymes are still needed to put the finishing touches to the prymnesin molecules.)
Big questions
A protein this huge poses several challenges. How does it fold to its native state within such a vast landscape of possibilities? The answer seems to be that each domain, linked to its neighbours via short chains, can fold independently. And how does such a large molecule, approaching the micrometre scale, fit within a cell? It might need an assigned location rather than simply floating in the cytoplasm, and there might be just one or two of them in each cell.
Identifying such a gene product stretches analytical methods to breaking point. One common technique, called poly-A RNA sequencing, captures the mRNA transcript of a gene by seizing the poly-A tail – a string of A nucleotides – that mRNAs generally have. But RNAs are physically fragile, and so a very large transcript will almost inevitably break during the process. What’s more, figuring out the structure of the protein from the sequence of its DNA is a delicate matter. If a single base pair gets misassigned, that creates a ‘frame shift’ that means all the subsequent codons (base-pair triplets encoding an amino acid) are misidentified.
Perhaps the hardest part of this problem is to shift our preconceptions. The size of the PKZILLAs, the researchers say, ‘expands our imagination on the capabilities of enzymes in the construction of complex molecules.’ To put it bluntly, you have to be open to the possibility in order to see it at all. That requires an acceptance that we still don’t necessarily know what the limits of molecular biology are. It’s almost as if the PKZILLAs recapitulate at the molecular scale the sort of biological recalibration demanded by the recent discovery of enormous bacteria: at around 1cm in length, individual cells of Thiomargarita magnifica are visible to the naked eye.3 Were these giant enzymes, like those bacteria found in a mangrove swamp, hiding in plain sight?
Could these big, modular enzymes be emulated or adapted for bio-inspired and bio-enabled synthesis of other complex molecules? Say you want to replace one functional group on the ladder-frame polycyclic backbone with another, perhaps to explore the pharmaceutical potential of derivatives. Could you just genetically engineer into the enzyme a slightly different module? Such engineering of PKSs has proved difficult – the control and specificity in these proteins may be rather fine-tuned. All the same, recent advances give cause for hope.4 Now the PKZILLAs show how much room there is to play with.
References
1 I Paterson and N Y S Lam, J. Antibiot., 2018, 71, 215 (DOI: 10.1038/ja.2017.111)
2 T R Fallon et al., Science, 2024, 385, 671 (DOI: 10.1126/science.ado3290)
3 J M Volland et al., Science, 2022, 376, 1453 (DOI: 10.1126/science.abb3634)
4 M F J Mabesoone et al., Science, 2024, 383, 1312 (DOI: 10.1126/science.adj7621)
1 T R Fallon et al., Science, 2024, 385, 671 (DOI: 10.1126/science.ado3290)
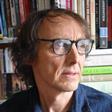
No comments yet