Political party conferences are not noted for taking much interest in science, says Philip Ball
The UK Conservative Party’s gathering in Manchester during the first week of October was something of an exception. The chancellor of the exchequer, George Osborne, visited the university to meet Andre Geim and Kostya Novoselov, the two scientists whose work on graphene won them last year’s Nobel prize in physics. He chose that occasion to announce that the government has earmarked £50 million to establish a Graphene Global Research and Technology Hub at Manchester - as Osborne put it, to ‘take this Nobel prize-winning discovery from the British laboratory to the British factory floor’.
Funding graphene research
It’s a rare bit of good news in these austere times for science funding. Osborne rightly hinted that the UK has long been poor at converting its impressive fundamental science into lucrative commercial products. Already, it seems that the first applications of graphene will come from non-British electronics firms such as Nokia and Samsung, who are thought to be planning uses of these strong, ultrathin carbon sheets in touchscreens for mobile devices.
Other proposed near-term applications include photosensors for optoelectronics, flexible yet strong graphene ‘paper’, and cheap high frequency electronic circuitry for radiofrequency detectors. Yet despite the hype, it’s far less certain that graphene, a semiconductor, will ever rival silicon as a substrate for the logic circuits at the heart of computers. Graphene’s band gap - the energy separation between its electron-filled valence band and its empty conduction band - is precisely zero. This means that it costs no energy for some electrons to cross the ‘gap’, and so unlike silicon, graphene’s electrical conductivity can’t easily be turned fully off. That would undermine the transistors needed for logic circuits, making them leaky and wasteful of power. There are ways of opening up a band gap in graphene, but not yet wide enough to make transistors comparable to silicon ones.
It might all sound like physics, but the key to turning the fundamental research into technologies that will repay such massive investment lies with chemistry. For one thing, patterning graphene into circuits might be best done from the bottom up: not by cutting up large sheets but by growing ribbons and junctions with atomic precision, as though they are giant organic molecules.1 Alternatively, Robert Haddon of the University of California at Riverside, US, reckons that circuits could be traced out by functionalising graphene to modify its electronic behaviour locally, making some parts of the sheet insulating, some semiconducting and so forth.2
Growing graphene
And while Geim and Novoselov famously made graphene by peeling off individual carbon layers from graphite using Scotch tape,3 it’s likely that the best and most reliable route to large-area films is chemical vapour deposition (CVD) of carbon onto a substrate.4 If that’s so, we’ll need to understand the chemical details. A new study by Stephan Irle and his coworkers at Nagoya University in Japan shows that these are not at all what one might expect.5 Their quantum chemical simulations of the deposition of C2 molecules on nickel indicate that, rather than forming graphene’s hexagonal mesh, the carbon assembles into a different, exotic and currently hypothetical form.
In 2000, nanocarbon specialists Humberto and Mauricio Terrones and their colleagues proposed that sp2-hybridised carbon could form flat sheets composed wholly of five- and seven-membered rings.6 Carbon pentagons introduce bowl-like (positive) curvature into a graphene sheet, and precisely 12 of them will allow such a sheet to close up on itself to form a hollow fullerene like C60.
Carbon heptagons, meanwhile, introduce saddle-like (negative) curvature. But in a mixture of the two, the positive and negative curvatures may cancel out, leaving the sheets flat. The Terrones brothers called such a structure haeckelite, because the ring network reminded them of the drawings of marine radiolarians by the German biologist Ernst Haeckel at the end of the 19th century. Radiolarians have biomineral exoskeletons made from a geometric mesh of hexagons, pentagons, heptagons and other rings.
Irle and colleagues found that the C2 molecules first condense into linear carbons chains, which then link to form haeckelite-like layers. These will gradually anneal to the more stable graphene over a timescale that is experimentally fast but longer than the 50-picosecond duration of the simulations. But starting from haeckelite could leave the sheets saddled with many non-hexagonal defects.7 If, however, a hexagonal ‘seed crystal’ is used to nucleate the deposition process, such as a ring of six fused benzenes
(a C24 coronene-like fragment), the hexagonal mesh grows from it right away.
The message, then, is that growing graphene by CVD might benefit from an initial injection of hexagonality to start off on the right footing.
References
1 J Cai et al, Nature, 2010, 466, 470 (DOI: 10.1038/nature09211)
2 E Bekyarova et al, J. Am. Chem. Soc., 2009, 131, 1336 (DOI: 10.1021/ja8057327)
3 S Chen et al , Nano Lett., 2011, 11, 3519 (DOI: 10.1021/nl201699j)
4 K S Novoselov et al, Science, 2004, 306, 666 (DOI: 10.1126/science.110289)
5 Y Wang et al, J. Am. Chem. Soc., 2011, DOI: 10.1021/ja2064654
6 H Terrones et al , Phys. Rev. Lett., 2000, 84, 1716 (DOI: 10.1103/PhysRevLett.84.1716)
7 G-D Lee et al, Phys. Rev. B, 2006, 74, 245411 (DOI: 10.1103/PhysRevB.74.214405)
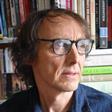
No comments yet