In search of design principles that would apply to living systems evolved anywhere in the universe
When Nasa’s Europa Clipper and the European Space Agency’s Juice missions reach Jupiter in 2030–31, one of their key objectives is to assess the habitability of the giant planet’s moon Europa, which has oceans of liquid water beneath its icy crust. But a big question is whether we’d know Europan life if we saw it, for life that arose and evolved independently from that on Earth could be reasonably expected to have a somewhat different chemical basis. But how different, really?
That’s the question explored in a new paper1 by an international team of researchers, several of whom are – like first author Ricard Solé of the Santa Fe Institute in New Mexico – experts on complex systems. Previously the question of alternative biospheres has been guided by little more than speculation. But Solé and colleagues seek to identify ‘design principles’ likely to be shared by all living systems.
Of course, the first issue here is what we consider to be living. Having just participated in an informal survey organised by biologist Michael Levin at Tufts University in Massachusetts, asking that question of a wide range of correspondents (including Solé), I’m left in no doubt that there is no consensus. However, the question can be sidestepped by looking at the entities we agree are alive – like us – and asking which of their characteristics are likely to be essential to any evolutionary process like the one that generated our biosphere.
We have no reason to expect alien life to use DNA and proteins
We might all agree, for example, that living systems must obey the laws of thermodynamics. In particular, they maintain their local organisation – a relatively low-entropy state – at the cost of increasing entropy in their environment, primarily by producing heat. That’s essentially what Erwin Schrödinger said in his 1944 book What Is Life?, but Solé and colleagues go further. To maintain a low-entropy state, living things must sustain chemical reactions that decrease entropy locally, which demands that they be coupled to some free-energy source, such as a concentration gradient. But organisms do this without the need for all reactions to have direct access to that source, by using molecular ‘energy intermediates’ such as ATP. And these reactions tend to be reversible so that organisms can store energy to buffer themselves against fluctuations in the source – the absence of sunlight at night, say.
Furthermore, biophysicist Harold Morowitz has shown that such non-equilibrium chemical systems must use cyclic reaction schemes,2 the most familiar being the citric acid cycle of metabolism. Already, then, we can draw some inferences about the kind of chemistry to look for in living systems.
It’s often (and rightly) pointed out that we’d have no reason to expect alien life to use DNA and proteins. But that doesn’t mean the molecular ‘information carriers’ can be arbitrary. Solé and colleagues say that there are reasons to suppose that linear, one-dimensional informational molecules are preferable to other geometries. The way information is read from a DNA strand looks remarkably similar to Alan Turing’s idea of a universal computer based on linear tapes of binary code, and there are good reasons to think that the read and write processes in such a case are simpler, most thermodynamically efficient, and easier to evolve than more complex methods based on, say, two-dimensional nets. This doesn’t mean that life itself really is a computation based on DNA, nor that all biological information processing is one-dimensional and digital (the 3D structure of chromatin matters too, for example), but it makes sense that the biological information carriers are linear polymers.
All known life is cellular (let’s defer viruses for now). Such compartmentalisation seems essential to allow a living system to be thermodynamically distinct from its environment and to maintain concentration gradients. What’s more, a closed compartment acts as a container to co-localise the various elements that John von Neumann argued are essential for a self-replicating entity: he proposed that the ‘constructor’ that does the building must be physically distinct from the ‘instructions’ it uses. Living things can’t be single molecules, nor unbounded collections of them.
Solé and colleagues also propose that there are universal constraints on multicellular life, on living things with higher cognitive complexity (which will be likely to have a particular neural network architecture to exhibit learning), and on ecosystems – for example, they will necessarily incur the problem of parasites such as viruses. In these respects, then, the researchers say that there may be a ‘logic of life’ that transcends both the chemical specifics and the historical contingencies of terrestrial evolution – a logic that can be inferred from the laws of physics and chemistry. Science-fiction writers might be dismayed by the implication that a life form such as that in astronomer Fred Hoyle’s 1957 novel The Black Cloud might not be viable. But (and here’s another puzzle) imagination is broader than the world.
References
1 R Solé et al., J. R. Soc. Interface Focus, 2024, 14, 20240010 (DOI: 10.1098/rsfs.2024.0010)
2 H J Morowitz, J. Theor. Biol., 1966, 13, 60 (DOI: 10.1016/0022-5193(66)90007-5)
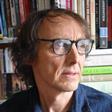
No comments yet