Researchers are searching for ways to replicate the self-healing properties of the ancient material
One of the perplexing aspects of the scandal about ailing concrete panels in British schools and other public buildings is that these components were only ever expected to perform for a few decades before they needed repair or replacement. The porous nature of the reinforced autoclaved aerated concrete (RAAC) at the heart of the problem makes it particularly vulnerable to deterioration, because water can more readily infiltrate and corrode the steel reinforcement. But even the best structures made from Portland cement concrete, the most common variety in use worldwide, are not expected to last longer than a century before needing maintenance.
That might be adequate given the typical rate of construction turnover in many modern cities. But it seems pitiful compared to the longevity of some of the structures made by the Romans. The concrete dome of the Pantheon in Rome remains stable enough for visitors to walk beneath, and some Roman harbours have underwater concrete elements that have not been repaired for two millennia – even though they are in regions often shaken by earthquakes.
Whence this remarkable resilience of Roman concrete architecture? It’s all down to the chemistry. The key component of mortars and cements is lime: calcium oxide, made by heating a calcium carbonate mineral such as limestone to drive off CO2. Add water and you have slaked lime Ca(OH)2, which was typically mixed as a paste with sand or rubble (aggregate) to make concrete. The hydroxide gradually absorbs CO2 from the air and reforms a hard binder of calcium carbonate.
But Roman concrete was different. They mixed lime with volcanic ash composed of glassy aluminosilicate minerals to make the cement that bound big chunks of volcanic rock in a kind of random three-dimensional mosaic. Instead of forming carbonate, the slaked lime reacted with components of the ash to produce calcium aluminosilicate phases – basically a kind of rock itself, which sets around the chunky aggregate in a similar way to how geological conglomerate rocks are made.
Better still, the concrete can go on reacting for years and even centuries. Water infiltrating into the material can dissolve calcium, aluminium and silicate ions from the volcanic material, which can then crystallise as hydrated minerals with plate or needle textures. These may reinforce the concrete by deflecting cracks, rather like the fibres in some tough composites. And if cracks do appear, the growth of the aluminosilicate crystals in the gaps seals them up again: the concrete is self-healing.1,2 According to Marie Jackson, a specialist in Roman concrete at the University of Utah, US, the volcanic ingredients ‘provide a reservoir of reactive material that can be mineralised over time to form new cementing material.’
That contrasts with a recent suggestion by researchers at the Massachusetts Institute of Technology in the US that the self-healing properties of Roman concrete were due to residual, microscopic pockets (clasts) of lime that could react with water permeating through cracks to form a calcium carbonate (calcite) sealant.3 That proposal remains controversial among experts; Jackson says that calcite is not a strong binding agent.
The Romans were lucky, because the volcanic ash (known as pozzolan) that they used, mostly collected from the region around Vesuvius near Naples, is not common worldwide. They shipped 20,000 tons of the stuff across the Mediterranean to build the concrete harbour at Caesarea Maritima in Israel, but that was hardly a sustainable proposition.
So Roman concrete fell out of use with the decline of the empire, and concretes returned mostly to the use of air-hardening lime until the 19th century. From around the 1750s, so-called hydraulic lime cement became popular, which contains clay and other silicate impurities so that it sets by hydration instead of carbonation and forms silicates rather than carbonate. But in 1824 a Leeds bricklayer called Joseph Aspdin patented a method for making a hard, fast-drying cement from slaked lime and clay mixed and baked in specific proportions.4 He cannily marketed it as Portland cement to capitalise on the popularity at that time of fine Portland limestone, used to build St Paul’s Cathedral and Buckingham Palace.
By the start of the 20th century Portland cement was the most common binder for concrete, and so it remains today. But some researchers are now seeking self-healing chemistry like that of Roman concrete using modern formulations: one approach has explored the use of recycled, pulverised silicate glass as an analogue of pozzolan.
Among other approaches to seal cracks against water ingress, one promising idea is to pepper the concrete with tiny capsules containing spores of bacteria that produce carbonate ions, leading to deposition of biomineralised calcium carbonate.5 If a crack splits a capsule open and exposes the spores to water, the bacteria are activated and seal the crack back up within a few weeks. It’s currently expensive and surely no panacea – but the RAAC crisis suggests that strategies like this might be more broadly useful than we’d thought.
References
1 M D Jackson et al., PNAS, 2014, 111, 18484 (DOI: 10.1073/pnas.1417456111)
2 M D Jackson et al., Am. Ceram. Soc. Bull., 2018, 97, 22
3 L M Seymour et al., Sci. Adv., 2023, 9, eadd1602 (DOI: 10.1126/sciadv.add1602)
4 K L Scrivener and R Snellings, Elements, 2022, 18, 308 (DOI: 10.2138/gselements.18.5.308)
5 I Justo-Reinoso et al, J. Build. Eng., 2021 42, 102834 (DOI: 10.1016/j.jobe.2021.102834)
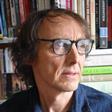
No comments yet