Experiments on battery electrodes and fuel cell catalysts while they’re being used – operando spectroscopy – can revolutionise our understanding of these crucial materials. Clare Sansom reports
- Definition and importance: Operando spectroscopy involves the simultaneous evaluation of the composition, structure and mechanism of materials while they are in operation. This technique is crucial for understanding and optimising the performance of functional materials like catalysts and battery components under real working conditions.
- Applications in battery research: The article highlights the use of operando spectroscopy in studying next-generation battery materials. Techniques like NMR and optical microscopy are used to observe changes in electrode materials during charge–discharge cycles, providing insights into lithium-ion transport, degradation mechanisms, and electrode heterogeneity.
- Catalysis and environmental impact: Operando spectroscopy is also applied to study catalysts used in processes like the hydrogenation of carbon dioxide. Understanding the structure and function of these catalysts under working conditions can help develop more efficient and sustainable chemical processes, contributing to the transition to net-zero emissions.
- Advanced techniques and facilities: The article discusses advanced operando techniques such as Bragg coherent x-ray diffraction and muon spectroscopy, which are used to study the dynamic properties of materials at synchrotron radiation sources. These techniques provide detailed insights into the structural changes and mechanisms of materials at the atomic and molecular levels.
Summary generated by AI and checked by a human editor
As the transition to net zero gets underway, more novel materials will be required for batteries and other forms of electricity storage, and as catalysts to convert waste products such as carbon dioxide into fuels and chemicals. Developing new functional materials, and improving existing ones, necessarily involves probing their composition, structure and function all the way down to the molecular and atomic level, and this often involves spectroscopy and microscopy techniques.
One of the ways of dividing up those analytical methods for studying materials at the micro- and nano scale that are grouped under the umbrella term ‘spectroscopy’ involves two main types. Experiments are classed as ex situ if a material is studied outside the device it is part of, or in situ if it is in contact with that device: if an electrode is kept within its battery during the experiment, for example. Furthermore, if that battery is functioning – charging and discharging – the experiment is not only in situ but also operando: a name taken from the Latin for ‘working’.
This term was first used in the context of catalysis in 2003 by Bert Weckhuysen of Utrecht University in the Netherlands and his colleagues. They defined it as ‘the spectroscopy of working catalysts, measured in realistic conditions of temperature and pressure’.
Operando spectroscopy can be defined as the simultaneous evaluation of composition, structure and the related mechanism of working materials. Currently, at least, the ‘working materials’ that are studied in this way are grouped into two basic categories: catalysts, and the materials that make up batteries and fuel cells. And the list of operando techniques that can be employed to characterise these materials in their working environments is a long one, including optical microscopy, optical (including infra-red and Raman) spectroscopy, NMR, fluorescence and muon spectroscopy among many others.
My interest in battery materials in particular was then sparked by meeting a scientist from Duracell
Clare Grey, University of Cambridge, UK
It is now impossible to imagine a world without lightweight rechargeable batteries. One of the most important properties of a battery is its energy density, defined as the ratio of the amount of useful energy stored in a system and its volume (or sometimes mass). The energy density of the now ubiquitous lithium-ion batteries, invented in 1991 and often used in the brick-like phones of the times, has trebled since the early 1990s while their price has fallen by 90%.
The concept of the lithium-ion battery is quite a simple one, although it involves some complex chemistry. The anode is most often made of graphite, the cathode is typically a lithium transition metal oxide – LiCoO2 was the first to be commercialised and is still in common use, although state-of-the-art cathodes often contain several different transition metals – and the electrolyte a stable organic liquid containing a dissolved lithium salt with high conductivity. Both electrodes are typically lithium insertion materials in which lithium ions can be introduced between layers of other atoms; these ions cycle through the electrolyte from the cathode to the anode during battery charging, and back to the cathode during discharge. The durability of such a battery is determined by the number of charge–discharge cycles that it can go through before the materials degrade.
A battery in the magnet
Clare Grey leads a group at the University of Cambridge in the UK that studies batteries and other electrochemical materials using analytical techniques including NMR and optical microscopy, both in situ and operando. ‘I began my research in NMR, and my interest in battery materials in particular was then sparked by meeting a scientist from Duracell at a conference who gave me my first battery materials and helped inspire a project for my third PhD student,’ she remembers.
Grey’s work now focuses on the structure and mechanism of materials for the next generations of rechargeable batteries and other electrochemical devices. These may be classified as either ‘post-lithium’, in which the chemistry is similar to that in lithium-ion batteries, but the lithium is replaced by another metal, or ‘beyond lithium-ion’, in which energy is stored without intercalation. She has been involved in the development of a prototype lithium–air battery, a beyond-lithium-ion device with a lithium metal anode and an ambient air cathode. ‘This is the only battery that has the same energy density as petrol, and it is therefore considered the ultimate battery for storing electricity,’ she says. ‘However, we have a long way to go before it will be practically usable.’
Jana Fritzke, a postdoc in Grey’s group, studies next-generation batteries and other energy storage materials using NMR. ‘NMR allows us to observe the atomic structures of solid and liquid phases at the same time, and if this is operando NMR, these structures can be observed while a battery is charging and discharging’, she explains. ‘But we have to run the battery while it is inside the NMR machine’s large magnet, and this presents an operational challenge.’ These experiments most often use the isotope lithium-seven, but many other NMR active isotopes are available, including carbon-13 and protium (1H) for redox flow batteries, sulfur-33 for lithium–sulfur batteries and oxygen-17 for lithium–air.
Not all operando methods visualise materials at the atomic level. Studying micro-structures that are large enough to observe through a light microscope, at what is sometimes called the mesoscale, can be equally important for understanding their properties. Alice Merryweather, a junior research fellow working in the Grey group, uses operando optical microscopy to observe changes in battery electrodes during charge–discharge cycles. Charging changes the electronic structure of electrode particles, and therefore their reflectivity. ‘We can see particles on the electrode surfaces getting brighter and darker in the microscope as their charge state changes and follow complete charging cycles with realistic timescales from just a few minutes to many hours,’ explains Merryweather. ‘This direct visualisation of local variations in state-of-charge provides new insights into lithium-ion transport, degradation mechanisms, and electrode heterogeneity.’
Grey and Merryweather have, together with physicists Akshay Rao and Christoph Schnedermann from Cambridge’s Cavendish Laboratory, founded a spinout company, illumion. The company is based around the development of a benchtop charge photometer device called the illumionONE, which is a microscope designed specifically for operando visualisation of charge changes in electrode particles during cycling. ‘I started my PhD research using a 2m × 2m optical table with components designed and built by hand,’ says Merryweather. ‘This compact device is far more convenient and easier to use.’ The company shipped its first device to a research institute in Germany in December 2024.
Big facility, small particles
Illumion’s charge photometer and even Cambridge’s powerful NMR instruments will often be much easier to access than the powerful, high-resolution ones available at synchrotron radiation sources, but there are properties of electrochemical materials that can only usefully be studied there. Two case studies from large national facilities illustrate how different operando techniques are being applied to transition metal oxide cathodes: one uses high-energy x-rays, the other a beam of particles generated in a neutron source.
Bragg coherent x-ray diffraction or BCXD involves smaller crystals than the micrometre- or even millimetre-scale ones in more conventional x-ray diffraction. While the conventional technique needs crystals that are large enough for the atomic or molecular lattice to be considered essentially infinite, BCXD produces distributions of x-ray intensity modified by a finite lattice shape rather than simple spots. That lattice shape can then be determined from the x-ray distribution using complex computational techniques.
Marcus Newton and David Serban of the University of Southampton in the UK used this method at the UK’s national synchrotron, Diamond Light Source in Oxfordshire, to study tiny nanocrystallites of the commercial cathode material LiCoO2. ‘This is a non-destructive technique that can probe defects in and distortions of a crystal lattice, and if it is operando, those distortions can be tracked in real time,’ says Serban, a PhD student.
The crystals Newton and Serban used were just about visible in a light microscope, similar in size to those typically used for powder diffraction experiments. The researchers monitored the stresses and strains on the crystals as a working battery was charged and discharged, observing how they changed with external factors. ‘We showed strained domains near the surface of the nanocrystals forming, expanding and fragmenting during charging, with the processes reversed during discharging,’ adds Serban. ‘This is an important reason for the loss of battery efficiency that is observed after many charge–discharge cycles.’
A much more complex layered transition metal oxide, LiNi0.8Mn0.1Co0.1O2 (known as NMC811), is showing promise as a cathode material, with a high base level of capacity (or energy density), but it loses too much of that capacity in the first charge–discharge cycle to be of practical use. Serena Cussen of University College Dublin in Ireland and her postdoc Innes McClelland are using operando muon spectroscopy at the Isis neutron and muon source near Diamond in Oxfordshire to explore the charge–discharge mechanisms in this material. ‘If we knew more about the reasons for this loss of capacity, we could design better cathode materials in the future,’ explains Cussen.
Muons are unstable negatively charged elementary particles that are very similar to electrons, but about 200 times heavier. They therefore penetrate further into materials than electrons, perturb their structures more, and can be used in spectroscopy. At the Isis source, an intense beam of muons is created by bombarding a thin carbon film with high-intensity protons, and when these muons are directed into a material, their precession and relaxation can be measured and used to determine properties of that material at atomic or near atomic-resolution.
The same source produces beams of high-energy neutrons, and these are more often used to measure the static properties of materials. In contrast, muon spectroscopy is most suited to measuring dynamic properties, so this technique has a particular value for operando applications. ‘When this technique is applied to the cathode in a cycling battery, muons will typically stop within about an angstrom of oxygen atoms, revealing important information about their immediate environment, its chemistry and the movements of lithium ions in the microseconds before they decay,’ adds Cussen.
Studying the future
The use of operando spectroscopy to understand, and hence to optimise, the properties of battery materials is currently the highest-profile application of this technology in the service of net zero, but it is not the only one. Weckhuysen emphasises the role of catalysts in what he calls ‘the refinery of the future’ in which hydrocarbons – which we expect to need for decades to come, for fuels, chemicals, coatings and plastics – can be synthesised from waste materials of carbon dioxide, plastic waste and biomass. The refinery of the future will need new and better catalysts for reactions like the hydrogenation of carbon dioxide to produce simple, harmless or genuinely useful products, and operando techniques will be employed to characterise them.
Operando spectroscopy is used to understand the relationship between structure, function and particularly mechanism in catalysts
Three of the beamlines at Diamond are dedicated to x-ray spectroscopy, including operando spectroscopy. The synchrotron’s large user community includes many scientists interested in probing the structure, function and mechanism of inorganic catalysts using operando techniques. ‘Our spectroscopists often study heterogeneous or solid–gas catalysts, where the catalyst is a solid and the reactants are in the gas phase,’ explains Sofia Diaz-Moreno, the group leader for these beamlines. ‘Operando spectroscopy is used to understand the relationship between structure, function and particularly mechanism in catalysts of the oxygen and hydrogen evolution reactions that produce molecular oxygen and hydrogen for energy storage devices,’ she adds. ‘We need to optimise the activity and selectivity of these catalysts to make them cheap enough to be economically viable.’
A much-needed update of the now 20-year-old Diamond synchrotron is now in hand, with the Diamond-II project expected to deliver new beamlines with greatly extended capabilities by 2029–30. Diaz-Moreno was involved in putting together the scientific case for the new Swift spectroscopy beamline, which, as its name implies, will extend the facilities for fast operando spectroscopy and studies of molecules in motion. ‘The high flux and high data collection rate of this new beamline will extend its ability to observe transient molecular species,’ she explains.
However, this expansion will come at a cost. The upgrade project was funded from July 2023, with the new facility expected to begin operation in 2029 and the first users expected on its flagship beamlines such as Swift from 2030. There is therefore a long lead time and in particular a long ‘dark period’ of about 22 months from December 2027, when the old light source is to be switched off, and June 2029 when the start of regular beamline commissioning begins. The impact of this on the user community is a worry for Diaz-Moreno. ‘Our spectroscopists have to do their experiments, they have PhD students and postdocs who need to build their careers … we need to make sure that they will have places to go to do their science, and that they come back to us when Diamond-II and Swift are operational,’ she says .
The net zero world of the future – or even of the mid-21st century – will depend on functional materials for batteries, catalysts and other devices, even more than that of today. Operando techniques, which have already transformed the field, will be essential for understanding these materials’ mechanisms of action and developing new ones. With so many of these techniques depending on synchrotron radiation in all its forms, the functional materials community will be watching for the end of Diamond’s ‘dark period’ with bated breath.
Clare Sansom is a science writer based in Cambridge, UK
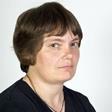
No comments yet