The decay and decomposition of a human body may be unpleasant to consider, but it can be crucial in criminal justice. Rupali Dabas talks to the forensic scientists developing techniques that can sniff out the truth
-
Stages of decomposition: The article outlines the stages of decomposition, starting with autolysis, followed by putrefaction and finally active decay. Each stage involves specific biochemical processes and the release of volatile organic compounds (VOCs) that provide crucial forensic evidence.
-
Forensic applications: VOCs released during decomposition help forensic scientists estimate the post-mortem interval (PMI). Techniques like headspace solid-phase microextraction and electronic noses are used to detect and analyze these compounds, aiding in criminal investigations.
-
Role of insects and microbes: Carrion insects, particularly blowflies, play a significant role in decomposition and forensic entomology. Their predictable development stages help estimate the time of death. Microbial communities also contribute to the decomposition process, with their succession providing additional forensic clues.
-
Research and body farms: Controlled environments like body farms are essential for studying decomposition. These facilities help researchers understand the complex factors influencing decay, leading to more accurate PMI estimations and advancements in forensic science.
This summary was generated by AI and checked by a human editor
It’s really difficult to hide a dead body. Decomposition leaves behind a wealth of forensic evidence – from organic compounds released by rotting corpses, to the distinct patterns of insect infestations. These tell-tale markers provide crucial insights for forensic scientists, helping them reconstruct the circumstances surrounding a person’s death. This field of study, known as forensic taphonomy, focuses on changes that bodies undergo after death and how they can help uncover vital clues to aid criminal investigations.
Decomposition begins moments after the heart stops beating, in a process called autolysis. Within minutes, cells are deprived of oxygen, and their acidity increases as by-products of chemical reactions accumulate. Enzymes break down membranes, releasing their contents into the surrounding tissues. This process starts in organs rich in enzymes, like the liver, and those with a high water content, like the brain. As all other tissues follow suit, damaged blood cells spill out of disintegrating blood vessels and, aided by gravity, settle at the bottom of the body, discolouring the skin in patches of lividity.
As carbon dioxide accumulates, it lowers the pH, creating an environment conducive to increased microbial activity, that go on to constitute the necrobiome. This marks the start of putrefaction – the second stage of decay – when the balance shifts from aerobic to anaerobic organisms that colonise the body. With the immune system out of commission, bacteria run rampant through the body, producing hydrogen sulfide and thiol-containing volatile organic compounds (VOCs), including methanethiol, dimethyl disulfide and dimethyl trisulfide, which contribute to a foul odour. These volatiles cause the body to bloat, which forces bodily fluids out of natural orifices or wounds. Noxious gases like indole, which smells like mothballs, and skatole, from faecal matter, add to the odour, creating a potent cocktail that also attracts insects. ‘VOCs can give us a lot of information about the state of the remains,’ explains Maiken Ueland, a forensic taphonomist at the University of Technology Sydney, Australia. ‘There are some key compounds that are present through the decomposition process; however, others change, and we can use this to develop methods to assist with determining time since death.’
VOCs can give us a lot of information about the state of the remains
The final stage is active decay. Bacterial action liquefies tissues, releasing cadaverine and putrescine, diamines produced during protein breakdown that generate the unmistakable stench of rotting flesh. These compounds are so distinct they are used to train cadaver dogs to locate human remains. ‘If we can understand what they are smelling we can make sure they are properly trained using the right training aids to improve them as a detection tool,’ says Ueland.
Understanding these stages of decomposition is more than just morbid curiosity. ‘By looking at the biochemical markers in the body, we use these to correlate time since death, or the post-mortem interval (PMI),’ explains Shari Forbes, a forensic chemist and taphonomist at the University of Windsor in Canada. ‘Since I do a lot of case work, I’m often asked to estimate time since death and it’s a real challenge.’ In some cases, an accurate PMI can mean the difference between proving innocence and identifying the guilty. Advances in forensic science, such as detecting the VOCs released during decay, are refining PMI estimates, helping investigators pinpoint timelines with greater precision.
Tracing death’s chemical signature
As the human body decays, it releases more than 400 VOCs, each conveying information about the decomposition process. Their emission pattern provides a useful method of determining the time of death, as the levels of different compounds fluctuate at distinct stages of decay. Sulfur-containing compounds, for instance, decrease over time, while nitrogen-containing compounds and alcohols become more prominent at later stages of degradation. This chemical fingerprint provided invaluable clues in a case involving two unidentified men, who were found dead in the sea near the Greek island of Samos in 2002. By analysing the VOCs present, investigators could pinpoint their respective times of death with reasonable accuracy.
VOCs also gained significant attention in Florida, US, in 2008 during the high-profile trial of Casey Anthony, accused of murdering her two-year-old daughter, Caylee. Prosecutors cited the distinct smell of human decomposition detected in the trunk of her car. Although chemical tests supported claims of substances typically found in decaying bodies, Anthony was found not guilty of first-degree murder and the case ignited a debate among forensic experts about the reliability of odour-based evidence in court.
Despite continual technological advances, relying solely on odour evidence remains challenging. Detecting VOCs early in the decomposition process is complex, which is why cadaver dogs often prove indispensable. ‘Dogs are the best search tool we have, but we’re always looking at other methods because you can’t always apply dogs,’ notes Forbes. Dogs can be costly to train, have limited working hours, and cannot operate in hazardous environments, which underscores the need for better detection strategies. And perhaps most crucially, occasionally they can be misled. ‘We found that some dogs indicated on chemicals not only associated with decomposition, but also with bodily functions like vomiting’, adds Anna Williams, a forensic anthropologist at the University of Central Lancashire in the UK. ‘It shows that there is a lot more research to be done on the full “bouquet” of VOCs from corpses, and of how trained dogs respond to them.’
To this end, researchers are refining field instruments to capture and identify VOCs more efficiently. ‘We are now able to get a lot more information about which VOCs are present than we did before with detection going from hundreds to thousands of compounds,’ says Ueland. One example is headspace solid-phase microextraction, a method that uses a silica fibre coated with a polymer, such as polydimethylsiloxane or polyacrylate, to absorb VOCs from the air above a cadaver. Gas chromatography then separates and identifies these captured chemicals. Another tool, sorbent tubes, works similarly by trapping VOCs in the air, either passively or using small air pumps. Portable devices like scent transfer units can also collect these odours on gauze or polyester materials, making them easier to analyse in the field or a lab.
In a collaborative effort, Forbes’ and Ueland’s labs have developed an electronic nose designed to mimic the scent-detection abilities of cadaver dogs. ‘Our e-nose work is pretty much at the forefront in terms of its application to human remains,’ explains Forbes. This e-nose can be used in locating missing persons or disaster victims by detecting and identifying VOCs, including alcohols, sulfides, nitrogen-containing compounds and hydrocarbons. Field trials have shown promising results, with the e-nose successfully identifying human remains hidden beneath debris in simulated disaster scenarios. To further refine the e-nose’s capabilities, VOCs are analysed using two-dimensional gas chromatography with time-of-flight mass spectrometry. This technique separates mixtures into individual components using two types of columns and then quickly identifies each component based on its mass. ‘We always use this because of the complexity of decomposition odour,’ explains Forbes. ‘It gives us far more information and puts us closer to understanding what the dogs are actually detecting.’
Where insects and bacteria take the stand
The chemical cocktail of VOCs is produced by microbial communities breaking down tissues, and serves not only as a signpost for forensic investigators but also as a powerful lure for insects. A rotting corpse is teeming with forensically important carrion insects drawn by the irresistible scents of dimethyl trisulfide and dimethyl disulfide.
Blowflies are the first to arrive, as they can detect a dead body from up to 16km away. Recognisable by their metallic blue and green sheen and loud buzzing, these land within minutes and begin laying eggs in any open wounds or natural orifices. What follows is gruesome yet essential: the eggs hatch into larvae or maggots that feed on the body, breaking it down and recycling nutrients back into the ecosystem. They then form hard pupal cases where they metamorphose into adult flies and the cycle begins again.
These insects, being cold-blooded, develop at a predictable rate that is directly influenced by ambient temperature. By identifying the oldest stage of insects present on a body and the species, forensic investigators can use local temperature data to estimate how long the insects have been present, and thus, how long the body has been deceased. ‘If we were able to find a body in Northern Manitoba where it’s really cold and we have maggots that are a month old… well they didn’t occur in January,’ says Gail Anderson, a professor of criminology at Simon Fraser University in Vancouver, Canada. ‘Those maggots must have developed before the insect season ended in October, which we know from the weather records.’ This predictable process has made forensic entomology, the study of insects in criminal investigations, an essential tool for solving crimes. ‘What I find the most fascinating is how smart flies are; we discovered while doing fieldwork was that flies would not lay eggs in the initial days if a recently deceased [body] has had a lot of chemicals in their system,’ adds Ueland. ‘It is like the flies know that the body is toxic, which is really impressive.’
One of the first recorded cases of forensic entomology comes from China in 1235, where a murder was solved by identifying the killer’s weapon from the presence of flies feeding on tissue and blood clinging to it. Since then, forensic entomology has become a cornerstone of criminal investigations, particularly in resolving the time of death. In 2002, when Kirstin Lobato was wrongfully convicted of the brutal murder of a homeless man, Anderson’s understanding of the timing of blowfly colonisation on cadavers was instrumental in showing that the murder occurred during a period when Lobato had an unchallengeable alibi. The body was found at around 10pm behind a dumpster on the Las Vegas strip in July 2001. The medical examiner initially estimated the time of death to be an hour or two before the body was found. But he changed his opinion several times, eventually settling on up to 24 hours before the body was found, within which Lobato would have had the time to commit the murder and flee the scene to justify her alibi. She was sentenced to 13 to 35 years in prison.
Some insects cannot feed on the body when it’s fresh
In 2009, Anderson was contacted to review the case. ‘They told me no insects were found on the body,’ explains Anderson. ‘If there are no insects, there has to be a reason for it, and it might be very straightforward.’ The pathologist originally stated he died just a few hours before he was found, ‘and I agreed with this because there were no insects. He probably died after it got dark because blowflies don’t fly at night. This time frame gave the young woman a very, very solid alibi and eventually we had a hearing December 2017. After spending 17 years in prison, she’s now free,’ Anderson says.
As microbes continue breaking down the body, the evolving VOCs cocktail influences the types of insects that arrive later. ‘Some insects cannot feed on the body when it’s fresh, but only when it’s been broken down a bit,’ explains Anderson. ‘They are not biologically capable of obtaining nourishment from it or they physically don’t have the mouth parts or the abilities to deal with tissue unless it’s got to a certain stage. So there’s a specific group of insects colonising the body over time.’ Beetles and other insects can only take over as the tissues dry out. The predictable succession of species provides forensic scientists with multiple biological clocks to estimate the PMI.
But insects don’t just provide answers about time. Their presence can reveal whether a body has been moved, disturbed, or exposed to specific environments, based on the types and sequence of insects present. A case in 2017 involved a 58-year-old woman found dead in her home in Brazil, initially declared to have died of natural causes. A later examination revealed blowfly larvae in an unusual location within the head. Unlike the typical colonisation of natural orifices, the larvae had accessed the head through a wound that was not naturally caused. This crucial detail prompted further investigation, leading to the arrest of a man who admitted to attempted rape, during which the head injury had occurred.
But the timeline of decay is not dictated by insects alone. ‘Post-mortem there will be a succession of microbes originated from the gut of the person, which is full of bacteria,’ explains Noemi Procopio, forensic scientist at the University of Central Lancashire, UK. ‘When a person dies, the body immediately becomes anaerobic, which is when the anaerobic bacteria in the gut colonise the whole body leading to the initial stages of bloating,’ she adds. ‘As the body ruptures, it becomes aerobic again, so there is another succession of bacteria which originate from the environment and from the soil, depending on the context.’
Flies and other insects bring their own microbiomes to the microbial communities on the decomposing carcasses. ‘This succession, despite the changes depending on the temperature, is overall quite predictable and has been shown to be consistent across rats, mice, pigs and humans,’ Procopio adds.
However, interpreting necrobiome evidence comes with challenges. If a body is moved after death, the sequence of microbial colonisation and species of insects present may no longer match the actual timeline, complicating efforts to reconstruct events. To address this, Procopio has launched an initiative that applies omics techniques to forensic science, aptly named ‘Forens-OMICS’. ‘We carry out deep and untargeted analysis of specific sets of molecules, integrating genomics for DNA, proteomics for proteins, and metabolomics for metabolites to analyse post-mortem changes at the molecular level,’ she adds. These analyses rely on biological material obtained from human bones or animal cadavers. ‘Where mapping modifications of DNA are great for the age of the person, and proteomics was more relevant for prolonged PMIs, metabolomics worked better on the shorter timescales.’ By combining these approaches to interrogate the microbiome, they are able to predict the PMI with increasing precision. ‘So now we are in that at that stage in which we need to identify which are the most promising biomarkers for each of these omics and when to recommend the use of one or another.’
Analysing the data generated by their high-throughput techniques is no mean feat. ‘We have a bioinformatics person in the group who collates the omics data and integrates them using machine learning approaches to generate models for PMI estimations,’ explains Procopio.
Once established, Procopio envisions these molecular markers to be crucial for accurately identifying PMIs, especially in cases where traditional forensic clocks are not decisive. ‘There were two individuals placed at a next to each other and left to decompose in a research facility on exactly the same day in June 2020. They had died at the same time and were exposed to the same environment, but after one day one body was noticeably more decomposed than the other and after 10 days, one was a skeleton and the other one was still entering early stages of decomposition,’ she notes. ‘By relying on observation alone no forensic taphonomist would have a know that they both died on the same day. But by doing other molecular analysis, this may be easier, so that’s our hope.’
Corpses to clues at the body farm
Given the complexity of decomposition, forensic scientists rely on controlled environments to study these processes in depth. ‘Decomposition is almost unique for each single person – we notice significant differences that we don’t yet know much about,’ says Procopio. Factors like location, climate, burial depth and scavenger activity can dramatically alter the stages of decomposition, making it challenging to pinpoint a precise time of death. Soil composition and burial conditions add further complexity, creating chemical signatures that often defy clear categorisation. ‘There is so much we don’t know about the influences, drivers and inhibitors of decomposition, that we need more robust, rigorous experiments using donated human cadavers to understand,’ adds Williams.
This unpredictability is why body farms – research facilities where remains are studied under controlled outdoor conditions – have become an indispensable resource for forensic science. In the UK, body farms primarily use animal cadavers, such as those at Wrexham Glyndŵr University’s facility. Animal cadavers provide valuable insights into how decomposition unfolds under different environmental conditions.
‘Most of the world cannot use human remains, so they use pigs in their local climate to obtain a more accurate representation,’ notes Forbes. ‘But if you want to study fingerprints, teeth or DNA, then you can’t use a pig. We have to use human cadavers.’ Human body farms are essential for bridging this gap, but there are currently none established in the UK. There are 11 across the world, with eight in the US.
‘For many, death and decomposition are a taboo subject, but once you get past the initial reluctance or hesitation, there is so much to discover,’ says Williams. The science of death extends far beyond academic curiosity. As Anderson emphasises, ‘we know that our research isn’t just an interesting thing that’s going to get us a publication. It actually means something. It could mean somebody’s freedom, somebody’s conviction. It means solving a crime.’
Rupali Dabas is a science writer based in London, UK
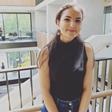
No comments yet