Failing to consider how solvent polarity affects the thermodynamic properties of photocatalysts is stopping scientists from accurately optimising their reactions, new research shows. ‘It would seem that up until now the concept of the effect of solvent had never been looked at,’ notes Eli Zysman-Colman from the University of St Andrews, UK, who led the work. Zysman-Colman’s team’s study also suggests that some photocatalysts are misclassified in the literature.
Photocatalysts are typically molecules that were originally developed for other applications such as organic LEDs. ‘A lot of people performing photocatalysis are synthetic chemists who are primarily concerned with optimising the yield of a transformation,’ Zysman-Colman tells Chemistry World. They often choose a photocatalyst based on optoelectronic and thermodynamic properties reported in the literature without reevaluating them under different conditions. So ‘the origins of why one works better than the other and [any] link to yield is tenuous because the underpinning photophysical data isn’t there.’
From now on, the solvent effect will be taken into account, and reported data will be more precise and reliable
Zysman-Colman and colleagues studied how eight photocatalysts – four phosphorescent emitters and four thermally activated delayed fluorescence emitters – behave in solvents of varying polarity (MeCN, DMF, DCM and THF). The study explored two types of photochemical reactions: photoinduced electron transfer and photoinduced energy transfer. Their original aim was to draw a correlation between solvent polarity and photocatalytic properties, and then reaction yield. However, they were unable to correlate any changes in the photocatalysts’ properties with yield because those involved in photoinduced electron transfer reactions were unstable. But they did establish that solvent polarity affects photocatalysts’ triplet energies and thermodynamic properties, altering their performance.
‘Finally, someone systematically studied the solvent effect in photoredox-catalysed reactions,’ comments Dorota Gryko, a photochemistry expert from the Polish Academy of Sciences. ‘From now on, the solvent effect will be taken into account, and reported data will be more precise and reliable. Furthermore, comparisons will be made only if the solvents are the same.’
Photoinduced electron transfer reactions
Photoinduced electron transfer (also termed photoredox catalysis) involves a single electron transfer between the excited photocatalyst and the substrate, where the reaction is either oxidative or reductive depending on whether the excited photocatalyst loses or gains an electron. On searching for a link between solvent polarity and yield, the team found that photocatalysts involved in photoredox catalysis photodegrade and their chemical properties change. Such degradation makes it difficult to determine which compound is really acting as the photocatalyst and made the group question whether they should even refer to them as photocatalysts.
Photocatalysis and photosensitisation are closely related and have similar Iupac definitions.2 ‘There is some confusion about this in the relevant communities,’ says photochemist Norbert Hoffmann, at the University of Strasbourg in France. ‘Generally, physical chemists prefer “photosensitisation”, while people working in the fields of catalysis or organic chemistry prefer “photocatalysis”,’ he adds. Given a catalyst’s properties should not change during a reaction, many in the community believe that if a photocatalyst photodegrades during photoredox catalysis it should instead be referred to as either a “photosensitiser” or “photoinitiator”.
Photoinduced energy transfer reactions
Photoinduced energy transfer often proceeds via a double electron exchange from the triplet excited state of the photocatalyst to that of the organic substrate. At present, researchers will deem a photoinduced energy transfer as thermodynamically allowed if the triplet energy value (ET) of the photocatalyst is higher than that of the substrate. However, Zysman-Colman’s team found that two photocatalysts with very different ET values had the same yield for the same reaction and that two photocatalysts with the same ET value had different yields. This implies that ET values do not capture all the information needed to judge a photocatalyst’s performance.
‘I think there are some really important lessons for the community to think about when they are trying to optimise reactions,’ Zysman-Colman adds. He hopes his team’s findings will prompt researchers ‘to be more reflective of what is going on in terms of thermodynamics as a function of environment but also [understand] that a “photocatalyst” is a species that can itself act as a substrate and in doing so can lead to other products that are themselves photoactive and so complicate the analysis.’
Eli Zysman-Colman’s advice for scientists optimising photocatalytic reactions:
- Remeasure the optoelectronic and thermodynamic properties of a photocatalyst when the environment of the reaction has changed.
- Check the photostability of the photocatalyst in photoredox catalysis, as this could impact the yield.
- Do not rely on the triplet energy value as the sole indicator of whether a photoinduced energy transfer reaction is thermodynamically allowed.
Correction: This story was updated on 13 March 2024 with Norbert Hoffmann’s new institution
References
1. M A Bryden et al, Chem. Sci., 2024, 15, 3741 (DOI: 10.1039/d3sc06499a)
2. C Michelin and N Hoffmann, ACS Catal., 2018, 8, 12046 (DOI: 10.1021/acscatal.8b03050)
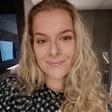
No comments yet