Scanning transmission electron microscopy experiments have uncovered the structural changes a ruthenium catalyst undergoes that increase its activity during an ammonia cracking reaction. The findings could help researchers design better heterogenous catalysts in the future.
Ammonia is often said to have more hydrogen than molecular hydrogen, explains Jesum Alves Fernandes from the University of Nottingham in the UK. This high hydrogen content and its ease of liquefaction mean many research groups are exploring ammonia’s potential as a carbon-neutral fuel and what materials perform best to catalyse its decomposition into nitrogen and hydrogen.
Various metal-based catalysts have been developed for ammonia decomposition and catalysts based on ruthenium appear to be among the most effective. Now, Alves Fernandes and colleagues have demonstrated how a ruthenium catalyst they have developed becomes more active with time and the single-particle changes that account for its performance.
The team prepared their catalyst using magnetron sputtering. ‘We realised that if we can manage the surface diffusion of metal atoms by controlling the barriers to atom movement, as well as factors like temperature, density and the nature of surface defects, we can design catalytic centres of desired size and shape,’ explains Alves Fernandes.
‘We do not use any solvents, reagents, ligands or other substances that might interfere [with any catalytic process]. As a result, our method is highly controllable, reproducible and even programmable. Additionally, no waste is generated, making this process ideal for large-scale fabrication,’ says Nottingham team member Andrei Khlobystov.
They then used identical location-scanning transmission electron microscopy to track the evolution of the individual ruthenium nanoclusters during a 20-hour-long ammonia decomposition reaction. ‘Each nanocluster undergoes internal changes, transitioning from amorphous shapes to crystalline nanoscale pyramids. These processes allow the catalyst to adapt to the reaction conditions and enhance its activity,’ says Khlobystov. The resulting pyramids have a large proportion of five-atom ruthenium clusters, known as B5-site-rich ruthenium nanoparticles, which are the active species in ammonia decomposition on ruthenium.
‘[The fact that the researchers] manage to follow the active sites at this scale, and the changes that improve its activity, is incredible. The catalysts are often dynamic and knowing what is going on at this level is crucial,’ comments Laura Torrente Murciano, a specialist in ammonia cracking and synthesis at the University of Cambridge in the UK. ‘Despite starting with a disordered ruthenium layer that is a poor catalyst, when the material is subjected to temperatures of 450°C under reaction conditions, reordering of the metal atoms happens, with an increase in activity.’
Khlobystov suggests that both the fabrication technique and the imaging technique they use could be applied more widely: ‘The imaging requires no extra specialist equipment other than the transmission electron microscope, which most institutes have.’ Torrente Murciano also says ‘other similar catalysts can be prepared using their technique – different areas of catalysis can all benefit.’
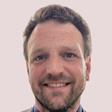
No comments yet