A study led by researchers at Purdue University in Indiana, US, has delivered a multifaceted overview of the contamination found in East Palestine, Ohio, and its surroundings following the 2023 Norfolk Southern train derailment. The team’s review of released data, alongside their own samples, highlights gaps in the official response to the disaster. It also reveals that chemical exposure continued for months following the derailment, despite the community returning to the area after just a few days.
On 3 February 2023, a train carrying a range of industrial chemicals derailed on the outskirts of East Palestine, a village with around 4700 residents. These chemicals spilled into the surrounding environment; unintentional and deliberate fires spread them further still. In the hours following the accident, a one-mile evacuation order was issued, affecting 1500–2000 residents. Authorities lifted the order on 8 February 2023 and reassured the community it was safe to return home . But soon after, locals, visitors and business owners began suffering from a range of health problems, including headaches, nausea and dizziness. This caused the community to raise safety concerns over the immediate and future health impacts of the disaster.
Three weeks after the derailment, a team led by Purdue University’s Andrew Whelton initiated a post-disaster study in response to the public outcry.1 They visited the area, talked to locals and set about sampling. Their aim: to better understand the chemicals present, their fate and human exposure pathways.
The team used a range of sampling and analytical techniques. They focused on rivers, buildings and commercial products, and selected their methods and target analytes according to the railcar chemicals and the limited information made publicly available about the authority-commissioned environmental testing. The team also replicated generic tests used by official responders, including total petroleum hydrocarbon screens. And to give a more detailed picture of the chemical make-up of contaminated areas, the team used analytical methods like GC–MS and ICP–OES to pinpoint specific organic and metal contaminants they thought might be present.
An overwhelming odour
During one of their first visits the team came across a commercial building with an acrid odour. Officials had already conducted authority-approved air-monitoring tests using photoionisation detectors (PIDs) at the premises after the evacuation order was lifted. The official datasheet recorded negligible contamination, but the contractor noted an overwhelming chemical odour, so strong that they needed to leave the building after just 10 minutes.
The business owner commissioned their own air quality tests around the same time as the official testing because of the smell and soot in their building. Their results reported butyl acrylate along with other volatile organic compounds (VOCs) from the disaster.
Suspicions surrounding indoor air quality prompted the Purdue team to test retail silicone wristbands stocked by the business. Silicone is an effective chemical sorbent for VOCs and semi-volatile organic compounds (SVOCs). Tests revealed several VOCs and SVOCs had contaminated the wristbands, including butyl acrylate, echoing the business owner’s tests.
Ten months after the incident, the authorities conceded that the PIDs were not sensitive enough to reliably detected harmful levels of butyl acrylate. The team could still smell the acrid odour on their final visit to the building four and a half months after the disaster. Exposure to the community had been continuous throughout this period.
To the team’s alarm, they discovered that not all the chemicals released in the disaster were being tested for in official air and water samples. During a review of the data released by the authorities, they spotted that ethylene glycol monobutyl ether acetate (or 2-butoxyethyl acetate) was included as a test compound, even though it was not disclosed as being released from the train or during the response. They also noticed that 2-butoxyethanol was conspicuously absent from the list. Official documents dated a week after the derailment list 2-butoxyethanol as a potential contaminant and the study notes that 25,000 gallons (over 90,000 litres) had been released during the incident.
2-butoxyethanol was overlooked until the team informed the authorities of their discovery. The team suspect similarities between the compound names caused confusion. ‘It seems like there was nobody that was doing quality control [or] quality assurance to make sure the statements that were being made corresponded to the underlying data,’ recounts Whelton.
The study concludes that the approval of handheld PIDs and the lifting of the evacuation order with inadequate air testing meant that people were exposed to chemicals. Whelton says ‘individuals making decisions about using certain sampling approaches and equipment did not understand the limitations of the sample approaches and equipment. And that’s a problem.’
The study also ascertained that water played a critical role in both pollutant transport and exposure, and that people in buildings located near contaminated creeks were likely the most exposed to chemicals. Contaminated water did not just damage life below the water line; it added to air and building contamination as pollutants flowed down creeks and volatised into the surroundings. Pollutant transfer into the atmosphere was intensified by using mechanical aeration units along the creeks without VOC capture, which at the time, the authorities cited as a way to promote natural breakdown of contaminants. While aeration can be beneficial to aquatic life, ‘if you blow VOCs in[to] the air … you’re going to smell something and it’s going to be an exposure,’ says Whelton. Locals identified a link between their proximity to aeration units and their health issues. In some cases, residents needed to leave their homes to reduce the symptoms they were suffering.
In light of their findings, the team recommend steps to limit the potential health and environmental impacts of a future disaster. ‘We really need people that are trained in understanding how to approach these complex issues in the initial stages of the disaster response,’ advises Whelton. The recommendations encourage transparency and cover the development of tools to predict chemical exposure as well as the introduction of formalised decision-making procedures. ‘The number of industrial accidents and natural disasters that prompt chemical exposure seem to be increasing, there is a need to more rapidly and thoroughly understand what chemical health threats should be addressed so we can better protect people from harm,’ adds Whelton.
While the study covers the months following the disaster, Whelton and his colleagues insist that human and environmental impacts caused by the incident warrant further investigation. This sentiment is shared by Diana Aga, an environmental chemist with expertise in chemical analysis from University at Buffalo, US, who says ‘it is an impressive work, but it is only the beginning of it’. ‘I think they have provided a lot of information that can be used a springboard for further studies.’
Recommendations from Whelton’s team on how to better protect human health and the environment before and after chemical disasters
Before
- Develop a formal approach for characterising complex matrices to provide initial chemical assessments.
- Create protocols for testing building exteriors for contamination.
- Design indoor air and surface sampling protocols to characterise contaminants that may settle in buildings.
- Improve spill response technologies to more effectively and safely remove volatile organic compounds from surface water.
- Create a model for predicting chemical emissions and resulting exposures from contaminated creeks and streams, with and without mechanical aeration.
- Develop evidence-based decision-making processes to protect workers and the public following chemical disasters.
After
- Identify all potential contaminant pathways and exposures.
- Characterise the raw materials spilled so that subsequent environmental testing can be optimised.
- Make test results public and provide an accessible explanation of their significance and limitations.
- Calculate the magnitude of chemical mass transfer for affected natural and built environments.
- Re-evaluate contaminant pathway and exposure assumptions based on population feedback and third-party evidence.
References
This article is open access
P B Coelho et al, Environ. Sci.: Water Res. Technol., 2024, 10, 3007 (DOI: 10.1039/d4ew00456f)
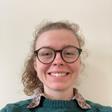
No comments yet