The detailed reaction mechanisms by which isolated hydronium and hydroxide ions neutralise each other have been observed by Israeli and Swedish research groups in ion-beam experiments. The results differ from what we expect to occur in liquid water, but could help guide future investigations into what is one of the most fundamental chemical reactions. The findings could also improve our understanding of the chemistry of the ionosphere and interstellar medium.
The random, spontaneous autoionisation of liquid water molecules into hydronium cations and hydroxide anions, coupled with their mutual neutralisation, is one of the most important equilibrium reactions in chemistry, underpinning acid–base equilibria. This is often accepted to proceed by stochastic proton transfer between water molecules. But it is not currently possible to study this experimentally because the autoionisation reaction is disfavoured by a factor of 107 relative to neutralisation, so one would need a huge sample.
A collaboration between researchers at the Hebrew University of Jerusalem, Israel and Stockholm University used the Swedish institution’s double electrostatic ion-beam storage ring cryogenic facility (Desiree) to merge hydroxide and hydronium ion beams, before detecting the neutral reaction products.
In this low-density environment, the researchers expected that most or all neutralisation would occur through electron transfer, as this can occur at larger interatomic distances than proton transfer. ‘Earlier neutralisation experiments were performed on atomic ions, so the proton transfer mechanism was not even considered,’ says Daniel Strasser, who led the project. The researchers did detect a predominant yield of electron-transfer products. However, they also found around two percent of the ions formed water molecules by proton transfer, which they believe to be a lower limit. The most common overall end products were a water molecule, a hydroxyl radical and a hydrogen atom. These could either be the result of an electron-transfer reaction producing a neutral hydronium radical that then falls apart or a proton-transfer reaction producing two water molecules, one of which subsequently splits.
The researchers believe their work could be important for studying reactions in Earth’s ionosphere and the interstellar medium, where hydroxide and hydronium produced by ionizing radiation have both been detected. Moreover, they could be used to refine quantum chemical simulations of liquid water. ‘We try to prepare our experiment in the same way that we can apply the best quantum chemistry simulations and to compare and to see if our understanding is correct, or if there is a place for improvements and corrections,’ says Strasser. The researchers hope that improved understanding of the isolated system will give theoreticians better insight into how to model both gas and liquid phases. The researchers are now studying the effect of replacing the hydrogen atoms with deuterium, and plan future experiments with ionic clusters that could bridge the gap to the liquid environment.
Experimental physical chemist Robert Continetti of the University of California, San Diego, says the work is ‘a very significant [study] on a very fundamental reaction’. ‘The value of this is as a benchmark for understanding liquid water, even if it’s a stretch,’ he says. Continetti agrees that the presence of proton transfer is a surprise and, when asked about possible further work, suggests – unprompted – studying deuterated water: ‘That could presumably reduce the contribution of the proton transfer because deuterium is twice as heavy as hydrogen and doesn’t tunnel as well.’
References
A Bogot et al, Science, 2024, DOI: 10.1126/science.adk1950
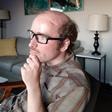
No comments yet