Interactions between neighbouring peptide bonds could explain how the collagen in dinosaur bones remains intact for hundreds of millions of years.
Bone collagen is commonly used for palaeobiological dating because of its deep roots in the animal kingdom – fragments of type-I collagen have been extracted from the bones of 68- and 80-million-year-old fossils of Tyrannosaurus rex and Brachylophosaurus canadensis, respectively. The longevity of peptide bonds in collagen is something of a chemical mystery though, as their half-life in a neutral aqueous solution is just 500 years, meaning they can persist for more than a million times longer than expected. A team based at the Massachusetts Institute of Technology (MIT) were intrigued by this longstanding puzzle and set out to find a chemical explanation for this surprising longevity.
‘Unlike most proteins, which are globular in structure, collagen is fibrous,’ says Ronald Raines, a natural products chemist at MIT and head of the lab carrying out the research. ‘It’s long, long chains that are wrapped into a triple helix and then those triple helix are packed against one another to make fibrils and ultimately fibres. Those fibres form the scaffold of the animal body.’
Raines, whose lab has been studying collagen for 25 years, says that it is possible to make very good models of collagen with peptides, which is useful, because each collagen strand is made up of 1000 amino acids. ‘That’s beyond the realm of synthesis, but it’s been known for 50 years that short peptides of only 20 to 30 amino acids will come together to form triple helical structures, just like those that are found in natural collagen,’ he explains. ‘That reductionist approach enables us to do experiments that would otherwise be extremely difficult.’
Molecular waterproofing
Using both experimental and computational tools, the researchers discovered a specific interaction – referred to as an n→π* interaction where ‘n’ is a lone pair of electrons and ‘π*’ is an antibonding orbital – that occurs between main chain acyl groups in neighbouring peptide bonds along the entire length of the collagen triple helix.
What makes these interactions interesting, Raines says, is that the Pauli exclusion principle means that two electrons cannot occupy the same orbital. ‘If this n→π* interaction enables donation from a lone pair n of one peptide bond into the n→π* orbital of the next peptide bond in the chain, then that orbital already has some occupancy,’ says Raines. ‘What we did was to develop an experimental test to see if this n→π* interaction that exists in collagen could protect the peptide bonds from attack by water, because if that π* was occupied by electrons from the neighbour in this n→π* interaction, then it can’t be attacked by electrons from water.’
They found that, apart for the three amino acids at the end of the chain, all 1000 amino acids in the three strands of collagen were protected by this interaction. ‘There’s no other structure that’s known that’s like that,’ says Raines. ‘That’s why we think it’s survived … it’s like a protecting group from organic synthesis, except it’s internal and it’s not a covalent bond, but it does provide this occupancy that fends off the water.’
‘Very exciting’
Mary Schweitzer, a vertebrate palaeontologist and evolutionary biologist at North Carolina State University, says that although the findings are ‘not unexpected’ they are ‘very exciting’. ‘Their approach is significant. For decades, it was thought impossible for molecules to persist … but, when more and more investigators began to truly look at ancient specimens, the impossible became probable, even likely. So, these authors took the next step, looking at mechanisms that could account for the “impossible” becoming observed with increasing frequency.’
However, she says that the limitation of their work is that their proposal is ‘hypothetical’. ‘Collagen is one of the best studied biomolecules on the planet, but their approach – to identify possible reactions on this molecule, driven by the inherent characteristics of the molecule itself – needs to be applied first to fossils themselves, then to other molecules.’
Schweitzer says that the characterisation of chemical modifications to the state of the living functional state, that could explain stabilisation, has been labelled ‘molecular taphonomy’ – taphonomy being the study of dead and decaying organisms. ‘The taphonomy of each molecule would be different and the environmental effects on each molecule would be as well,’ she adds. ‘Collagen in a desert fossil might have different kinetics than one from a deltaic environment.’
‘It would be great to characterise the reactions of collagen from different specimens – that would be a natural follow-on to this study. They haven’t actually looked for these components from fossil bone, or even from extant bone, that I can tell. But it is a good start, for sure. Their work should inform other studies, as well as stimulate additional work in molecular palaeontology.’
She adds that the work has ‘tremendous implications’ for research on ageing, drug design and molecular evolution. ‘Understanding environments that favour such preservation is also crucial to further molecular studies on fossil bone. Dinosaurs and other ancient organisms lived and thrived in environments very different than our own and how they adapted is, after all, a molecular question.’
References
J Yang et al, ACS Cent. Sci., 2024, DOI: 10.1021/acscentsci.4c00971
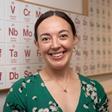
No comments yet