Over a quarter of hypothetical tricyclic benzene isomers that earlier computational studies had classed as stable are completely unsynthesisable due to quantum tunnelling.1 That’s according to quantum chemistry calculations by Sindy Julieth Rodríguez and Sebastian Kozuch from Ben-Gurion University of the Negev in Israel.
Benzene has been a hot topic of discussion among chemists since the early 1800s, with its strained isomers such as Dewar benzene and prismane leading to the discovery of aromaticity and resonance theory. In 2001, Gopalpur Nagendrappa, of Bangalore University in India, put forward 217 possible isomers of benzene.2 ‘We only know five of these structures have been synthesised,’ says Kozuch. ‘There are 1000s of other structures that nobody dares to synthesise or to start developing techniques to prepare them.’ Given benzene’s significance in organic chemistry, it is surprising that a reason has not yet surfaced as to why so many hypothesised isomers have not been isolated.
Now, Rodríguez and Kozuch have identified that for tricyclic benzene isomers, at least, quantum tunnelling could be partly to blame. Quantum tunnelling is when quantum particles, like atoms or electrons, overcome a potential energy barrier that they should not have sufficient energy to cross. Kozuch explains that ‘very strained molecules, very strange molecules,’ like tricyclic benzene isomers, are very reactive and often follow exothermic degradation pathways that leave them vulnerable to quantum tunnelling. Computational studies, however, rarely consider quantum tunnelling effects.
Rodríguez and Kozuch therefore decided to adapt computational quantum chemistry calculations to consider nuclear quantum effects and used them to analyse the molecular stability of 73 predicted high-energy tricyclic isomers of benzene. By studying the half-life of the isomers at temperatures close to absolute zero it meant that kinetic energy would be negligible and quantum tunnelling would be the only remaining mechanism at play.
Sure enough, degradation pathways generated by the calculations revealed that 19 supposedly stable isomers experienced quantum tunnelling instability.
Quantum tunnelling typically involves hydrogen atoms, but more and more reactions are being found to exhibit heavy atom tunnelling, involving atoms such as carbon. ‘We determined that most quantum tunnelling decomposition reactions [here] will occur by the breaking of the longest carbon–carbon bonds,’ says Rodríguez. A carbon–carbon bond length of 1.58Å, they found, generally corresponded to an unstable isomer.
Further to uncovering these unstable isomers, analysis of the isomers’ kinetic stability in classical and quantum regimes revealed other unusual behaviour. One isomer showed tunnelling control, where it degraded via one of two possible pathways depending on the temperature of the system. Another isomer exhibited domino tunnelling, where it degraded twice by quantum tunnelling.
‘This [study] should be a model for those people studying gas-phase chemistry and should encourage people to look into heavy atom tunnelling’ when working with strained molecules, comments Cina Foroutan-Nejad, a theoretical and computational chemist from Polish Academy of Sciences.
Foroutan-Nejad adds that the most beautiful part of Rodríguez and Kozuch’s study ‘is that something so simple like benzene, which has been known for one and a half centuries, maybe even more, still has enough space to be studied in more detail.’
References
1. S J Rodríguez and S Kozuch, Chem. Sci., 2024, DOI: 10.1039/d4sc05109b
2. G Nagendrappa, Reson., 2001, 6, 74 (DOI: 10.1007/BF02839086)
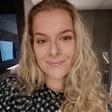
No comments yet