Nuclear batteries can provide long-lasting power to pacemakers, sensors embedded in buildings and bridges, and even planetary rovers. While the technology has existed for decades, recent advances mean that many research organisations and companies are now exploring a range of new opportunities to grow the batteries’ use in commercial applications.
These micronuclear devices harness the energy released during the natural decay of radioactive isotopes, but are much smaller than any nuclear reactor. In general, nuclear batteries produce just microwatts or even nanowatts of electricity. ‘The level of power these things can produce is way, way lower than if you’re [splitting] the atom,’ says Michael Rushton, a materials scientist at Bangor University in the UK.
Nevertheless, this small – yet reliable – energy output still makes them suitable for certain applications like powering sensors, especially under extreme temperatures or in locations with no available sunlight. The long lifespan of these batteries is another advantage, ranging from decades to hundreds of years or more.
‘You could embed a tiny nuclear chip with a sensor into concrete, come back a decade later and query the sensor about humidity on certain days,’ says Peter Cabauy, chief executive at City Labs, a Florida-based company developing micronuclear batteries. ‘Put it down. Forget about it. Use when necessary.’
Alphas-decay batteries
Nuclear batteries can be based on a range of different radioisotopes and each of these brings different advantages and challenges. ‘The alpha-decay radioisotopes possess a higher decay energy,’ notes Yaxing Wang at Soochow University in Suzhou, China. He was part of a team that recently reported a dramatic boost in the efficiency of converting americium-243 radioisotope decay into usable electrical power.
A standard design for micronuclear batteries based on alpha decay involves two key layers: a radiation source, and a scintillator made of a radioluminescent material that releases photons when it is bombarded by alpha particles. These photons then drive a photovoltaic cell to produce an electric current. But in Wang’s new setup, the radioisotope is incorporated alongside a terbium-based transducer unit within a single crystalline material.
‘The decay energy of α-particles from the radioisotope can be very efficiently deposited on the neighbouring high-impedance transducer unit at distances on the Ångström scale, minimising the unwanted energy loss,’ Wang explains.
As a result, the new design is able to convert alpha-decay energy into light 8000 times more efficiently than a setup based on separate americium and terbium layers. Overall, Wang’s radiophotovoltaic battery produces twice as much power per decay event than the previous record-holder, a battery based on the alpha decay of plutonium-238 that was developed 16 years ago.
In a classic classroom demonstration, alpha particles can be blocked with material as thin as a piece of paper. This means that shielding batteries based on alpha-decay is relatively easy. In the past this allowed plutonium-238 nuclear batteries to power pacemakers, until they were superseded by lithium-ion batteries.
Nevertheless, plutonium-238 remains popular for applications in space exploration. With its half-life of 88 years, it has been used as a heat source on more than two dozen Nasa missions. ‘Put a cup over a pellet of plutonium-238 and it will start to glow red,’ says Rushton. ‘If you couple that to a thermoelectric system that converts heat into electricity, you’ve got a battery.’
Batteries based on this design were used to power Nasa’s Voyager 1 space probe that was launched in the 1970s, and even the much more recent Perseverance rover, which landed on Mars in 2021.
However, Rushton notes that access to plutonium-238 is complicated as it is a controlled material and, typically, a research reactor is needed to make it. ‘A lot of those have been closing down in recent years,’ he adds.
Betavoltaic devices
City Labs focuses wholly on tritium, a radioisotope of hydrogen that emits beta particles. In contrast to the specialist uses of alpha-emitters, batteries based on beta-emitters are much closer to being used in commercial devices.
These betavoltaic devices act similarly to photovoltaics. However, instead of photons hitting a solar material, beta particles bombard a semiconductor and knock out electrons, creating electron–hole pairs. A charge difference builds up between the semiconductor’s positive side with an excess of holes (p-type layer) and its negative side with an excess of electrons (n-type layer).
‘The operation of betavoltaic cells is simple in concept, but getting the junction to work just right in practice with betas is difficult,’ says Cabauy. The bandgap of the semiconductor must be tuned to the energy of the electrons released by the beta emitter.
One benefit of using tritium as a radiation source is that it is produced in Candu heavy-water reactors, meaning that there is a ready supply of the material for nuclear-battery developers to use.
‘We know there’s scalable production of tritium,’ says Cabauy. ‘We’ve talked to national labs and companies that are showing the path forward to make more.’ Another advantage is that other hazardous radioisotopes are not made alongside tritium and it decays into the relatively safe and stable helium-3 isotope.
Tritium’s half-life of around 12 years gives City Labs’ batteries a lifetime of at least 20 years, producing nanowatts to microwatts of power per square centimetre. While it might sound tame, these tiny quantities of power have become more relevant in recent years. ‘When we started out, people said of nanowatt and microwatt power: “That sounds like static electricity,”’ says Cabauy. ‘Now clever engineers and scientists are finding ways to harness that.’
Tech race heats up
However, tritium is not the only game in town. The beta-emitter promethium-147, one of the main isotopes produced in fission reactors, has attracted interest over many decades and was used in the 1970s to power pacemakers. And earlier this year, Betavolt in China announced a battery based on the beta-emitting radioisotope nickel-63 and a diamond semiconductor, which it said would be the first mass-produced nuclear battery
Nickel-63 is typically produced inside a nuclear reactor and has a half-life of around 100 years, decaying to the stable isotope copper-63. Betavolt claims that its coin-sized battery generates around 100 microwatts of electrical power and has a lifetime of 50 years.
Meanwhile, a group at the University of Bristol, UK, is extracting carbon-14 isotopes from irradiated graphite from former nuclear power plants. Tom Scott and his colleagues incorporate this long-lived radioisotope into lab-grown diamond to generate current. In 2020, the Bristol researchers founded Arkenlight to develop such a battery and unveiled the first ever carbon-14 diamond and diode devices in September this year.
‘They’ve been turning the irradiated graphite into diamond film, which itself is a wide-band gap semiconductor,’ says Rushton. ‘You’ve got diamond made from a beta emitter, which irradiates itself and creates electricity. That’s neat.’ Since carbon-14 has a half-life of 5730 years, the batteries could provide intermittent power for thousands of years.
It’s these extended lifetimes of nuclear batteries that make them particularly well-suited for applications like space exploration, and long-term monitoring of infrastructure or challenging environments. ‘Such applications could include deep-sea exploration, space missions or remote monitoring stations,’ says Wang.
‘We’ve got our tritium chip and our sensors. We could drop them down an oil well and leave it there working away to monitor for leaks,’ adds Cabauy.
Brian Ellis from Canadian Nuclear Laboratories highlights the particular advantages that nuclear batteries offer for space applications. One day on the moon lasts around 29.5 Earth days, and for half of that time the surface is in dark. Temperatures during the day can hit 120°C near the equator; in contrast, deep lunar craters can slump to -246°C. ‘Radiation is always emitted. It doesn’t matter if it is hot, its cold, or if the sun is shining or not,’ says Ellis.
City Labs is working with Cornell University on tritium chips to power independent tracking units on low-earth orbit satellites. This could keep tabs on a satellite even after it stops working. Cabauy has also proposed that Nasa use them to power sensors in dark lunar craters. ‘For probes going to Mars or the moons of Jupiter, there’s a need for isotopes that can supply low power, consistently,’ he adds.
He also notes that the company is also actively working on using tritium to power pacemakers and is in talks with a US manufacturer.
Due to the fact that the radioisotopes used to power nuclear batteries are generally produced in nuclear reactors, micronuclear battery innovation has historically been driven by national nuclear labs, particularly in the US, Canada, Russia and China. And projects tend to remain within borders, given the nature of the material.
Nonetheless, Scott observes that recently interest in micronuclear batteries has ticked up. ‘Numerous governments [and] research organisations are all taking an active interest in this technology,’ he says. ‘We appear to have something of a technology race on our hands.’
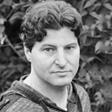
No comments yet