Make everything a bit bigger. That’s the general idea behind a quantum simulator that has been used to make analogues of small organic molecules. Bigger means more control. And more control means more answers.
Quantum simulators based on quantum mechanical systems let scientists study model systems and then extrapolate from them to understand real (and not-yet-real) systems. By using a quantum device rather than a classical computer, you’re not hindered by the computational costs that grow exponentially with every extra atom or the compromises that come with trying to model quantum.
Richard Feynman first floated the concept of building experimental platforms out of elementary quantum particles, like atoms, ions and photons, for studying many-body systems in his 1981 lecture Simulating physics with computers. ‘Nature isn’t classical, dammit, and if you want to make a simulation of nature, you’d better make it quantum mechanical, and by golly it’s a wonderful problem because it doesn’t look so easy,’ he said. His original idea involved building a lattice of spins with tuneable interactions.
In the years that have followed, analogue quantum simulators – quantum systems made to explore specific problems in quantum physics – have been made using trapped ions, cold atoms in optical lattices, liquid and solid-state NMR, photons, quantum dots and superconducting circuits. They’re subtly different from quantum computers that combine classical and quantum computing, and are being developed to accurately model chemical systems, among other things.
Last week we reported on a new quantum simulator. It’s made by placing rings of caesium ions on an indium antimonide substrate using a scanning tunnelling microscope. Each ring of caesium ions acts as an artificial atom, and when six of them are placed in a hexagonal shape they create an artificial version of benzene. Having established that the orbital patterns in this artificial benzene resemble the real thing, the team went on to explore more unstable systems such as cyclobutadiene. What’s particularly exciting about this approach is that by allowing users to make structures with fragile low-energy states, they can unpick the relationship between geometry and electronic structure. This new quantum simulator also stands out because it manages to remain uncoupled from, and therefore uninfluenced by, the supporting substrate.
An obvious challenge for quantum simulators, however, is verifying their accuracy. Luckily several teams have been developing techniques to do just that. As quantum simulators become even more sophisticated, it’s important that their trustworthiness and falsifiability keeps pace.
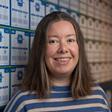
No comments yet