New wave of precision medicines amplify or silence genes, without altering genetic code
A new class of medicines that control the activity of individual genes could transform the treatment of numerous diseases, from viral infections to neurological disorders. The first human trial of a therapeutic based on an ‘epigenetic editor’ began earlier this year, with a string of others due to start in the coming months.
Over the last decade or so, tools like Crispr that allow precise targeting of specific sections of DNA have led to a plethora of medical treatments based on gene editing. These therapies generally cut DNA at specific points, allowing for faulty genes to be repaired or replaced.
Now, several genomic medicine companies are developing treatments based on a similar, but subtly different, concept. In contrast to gene editing, epigenetic editing doesn’t change the DNA sequence. Instead, epigenetic editors add or remove chemical tags to genes that regulate how much those genes are expressed. The technique mimics the natural mechanisms that cells use to turn different genes on and off.
The basics
Epigenetic changes happen naturally throughout our lives and in response to environmental stimuli. There are several different ways in which they alter a gene’s activity. For example, adding methyl tags to a gene can make it less accessible to the ribosome – the cell’s machinery that reads DNA and enables the production of the protein that each gene codes for. As a result, a methylated gene will become less active, or even silenced altogether.
Acetyl tags can make it more likely that a gene is read by the ribosome, meaning that the protein that the gene codes for will be expressed in greater quantities.
Activation of some genes and not others explains how distinct cell types are produced, despite the DNA stored in each cell containing the same full set of genes. But while epigenetics is an essential part of biology, dysregulation of these mechanisms is linked to many diseases. The new epigenetic editors that are now entering human trials aim to tackle this in a targeted and site-specific way.
Any off-target effects that an epi-editor makes are very, very unlikely to have any significant impact on biology
Derek Jantz, chief scientific officer at Tune Therapeutics, explains that while the potential of epigenetic editing was recognised decades ago, recent technological advances have finally made it a realistic proposition. ‘We have Crispr–Cas that we can use to very easily reprogram these things to hit different locations. We have a much better understanding of non-coding regions of the genome and how they affect gene expression. We have delivery technologies that didn’t exist until recently,’ he says. ‘So everything, just in the last couple of years, has come to a head that now it is possible to do this, and we’re seeing a small number of programs moving into the clinic.’
Researchers investigating these potential treatments point to numerous advantages. For example, epigenetic editors simply alter the expression of existing genes, rather than cutting and introducing permanent changes to the DNA sequence. And because an epigenetic editor needs to add multiple tags to the DNA to significantly alter gene expression, the consequences of an off-target hit with an epigenetic editor are unlikely to be as drastic as with a gene editor.
‘If you think about gene editing or base editing, you can imagine Crispr–Cas floating through a cell, it hits the genome in the wrong place, it cuts the DNA, it introduces a mutation into the DNA, and that mutation is there forever. It’s a binary effect – the gene is either edited or it’s not,’ says Jantz. ‘That’s not the case with epigenetic editing. The way epi-editing works is much more stochastic.’
‘Using methylation, for example, if we target a DNA methyltransferase to a particular region in a gene, and it adds a single methyl group to a single base in the genome, that is very unlikely to have any effect whatsoever. And in fact, it’s probably going to get lost as soon as that cell divides, as soon as the DNA is replicated,’ he adds. ‘So what has to happen is that methyltransferase has to hit that site over and over and over again, and it gradually builds up methylation … and only with repeated hits from the methyltransferase do we build up enough methylation that the gene actually gets silenced.’
Modern genome-wide RNA-sequencing assays enable companies like Tune to quantify whether an epigenetic editor has made any unwanted changes to gene expression. ‘If they are there, we can find them,’ says Jantz, but generally there are few, if any, he adds. ‘So it is a much, much easier argument to make to regulators, investigators and patients, that the off-target effects that an epi-editor makes are very, very unlikely to have any significant impact on biology.’
In the clinic
Tune, which has US sites in Durham and Seattle, was launched in 2021 to build on the research of Duke University’s Charles Gersbach. The company just raised $175 million (£140 million) of investment, which it says will enable it to develop its technology platform and expand its pipeline. Importantly, the money will also support its clinical program: in November 2024, Tune received approval to start a clinical trial of its hepatitis B (HBV) treatment in New Zealand. A similar trial in Hong Kong was approved in January.
‘We picked those two sites in part because they have a very high prevalence of chronic hepatitis B, but also because the two most prominent HBV clinical investigators are in New Zealand and Hong Kong. We were very fortunate that these two individuals, M F Yuen and Ed Gane, saw the potential for our approach and wanted to be the investigators on the study,’ explains Jantz. ‘So that is underway now, and we are getting patient data in and looking to see what it does.’
Tune’s treatment is delivered by a lipid nanoparticle to hepatocytes in the liver, where the hepatitis B virus replicates. Within the nanoparticle is mRNA that codes for a protein that induces epigenetic changes to the viral genome. ‘We silence the virus, both through methylation and through changes in chromatin structure, and that then turns the virus off,’ explains Jantz.
One benefit of the approach is that the effects on the virus persist after the therapy has been cleared from the body. ‘It’s a completely different way of thinking about HBV treatment, in that you don’t have to constantly redose to keep the virus suppressed,’ says Jantz. ‘We should be able to do it with a single shot, or a limited number of shots. You can imagine coming in, treating a patient once, suppressing the virus to 90–99%, coming in again maybe two or three times, and getting it all the way off.’
Delivering the goods
Another company looking to initiate clinical trials with an epigenetic-editor-based treatment for hepatitis is nChroma Bio. The company, based in Boston, US, is currently carrying out preclinical studies on its lead candidate, and recently secured $75 million to move the programme forward.
‘We’re going to be filing a [clinical trial application] for this year, which is in hepatitis B and D.’ says nChroma Bio’s chief scientific officer, Melissa Bonner. She highlights the huge opportunity of a successful therapy, given a general lack of treatments for both hepatitis B and D, and the fact that hepatitis D increases the risk of developing liver cancer.
‘It also represents an interesting target, because it doesn’t actually exist in the human genome, so the specificity for the hepatitis genome of the epigenetic editors is pretty exquisite,’ she adds. ‘So we’re seeing very specific targeting to the hepatitis genome in our preclinical models. We’re seeing robust silencing of the hepatitis genome, and we’re seeing [almost 99.99%] reduction of hepatitis B surface antigen.’
The chemistry coming out from our genes is written in epigenetics – it’s really the regulatory layer of the DNA
nChroma Bio was formed in late 2024 by a merger of Chroma Medicine, which specialised in epigenetic medicines, and NVelop, which developed new drug delivery technologies. Like Tune, nChroma’s hepatitis treatment is currently delivered inside lipid nanoparticles. However, by combining the two companies’ expertise, nChroma believes it will be able to develop medicines that use more complex delivery systems, to access harder-to-reach tissues.
Bonner says the company plans to exploit virus-like particles to target tissues beyond the liver, unlocking cell and tissue types that are currently really challenging to access. ‘That way we can actually get these amazing cargoes … to places, so that they can have therapeutic benefit – I think that there are definitely use cases where epigenetic silencing in combination with targeted delivery in vivo is going to be something that we’re very interested in and actively developing.’
Further indications
San Francisco-based Epicrispr Biotechnologies (Epic Bio) is aiming to launch a trial of an epigenetic editor delivered in a viral vector this year. The company was founded in 2021 by Lei Stanley Qi, who eight years earlier developed a way to silence genes using a ‘catalytically dead’ cas9 protein, while working in the lab of the Crispr pioneer Jennifer Doudna.
Epic Bio has raised $68 million to begin a human trial this year of a treatment for facioscapulohumeral muscular dystrophy (FSHD), a heritable disease that severely weakens numerous muscle tissues. The disease is linked to expression of a gene called Dux4. Usually, this gene is only active in young embryos, before natural methylation turns it off. But in FSHD patients, the gene is undermethylated, leading to production of a protein that is toxic to muscle cells. Epic Bio’s approach is to use an epigenetic editor to add more methyl groups and silence the gene.
‘It’s becoming clear that this particular gene in the muscular cells – human myocytes – is expressed in abnormally high levels in patients … leading to high toxicity. And this gene has multiple copies, so [gene] editing is not a solution,’ says Qi. ‘The key is to moderate with epigenetics to restore it to the normal level.’
One of the unique features of Epic’s platform is the CasMini system that was developed in Qi’s lab at Stanford University, US. CasMini is less than half the size of Cas9, which makes it easier to package into viral vectors that are, so far, the only clinically proven way to deliver therapies to harder-to-reach tissue types.
‘So if you want to get to the skeletal muscle as an example, the only way people have been able to deliver [drugs] right now is with [a viral] vector, ’ explains Epic Bio chief executive Amber Salzman. ‘When you use an [adeno-associated virus] to deliver to the muscle, that payload is actually pretty small – so cas9 won’t fit,’ she adds. ‘This is where – building on Stanley’s CasMini – we open up the door to diseases [in many other] tissue types.’
Epic Bio has now received approval for a human trial of its FSHD therapy in New Zealand and the US. Salzman explains that the company’s trial will initially involve three patients. ‘It’s more of your typical … gene therapy trial, which usually starts at the minimally efficacious dose,’ she says.
If the drug is well tolerated, the trial will take on two further groups of three patients, gradually increasing the dose. ‘We’ll be looking not just at safety, but a few things,’ adds Salzman. ‘One is we’ll be doing a muscle biopsy at baseline and month three – and at month three, we’ll be able to see: do we, in fact, methylate? Do we suppress the downstream of Dux4, as well as Dux4? So it’ll basically prove that we have target engagement. And then of course, we’ll also be monitoring functional measures like muscle strength and stride velocity.’
Targeting the CNS
Sangamo Therapeutics, headquartered in Richmond, US, is another company aiming to solve the challenge of delivering epigenetic editors to different tissue types. Rather than a Crispr–Cas based system, Sangamo’s platform is based on zinc-finger proteins that recognise and bind to DNA. The small size of the zinc fingers means that they are easier to fit into viral vectors.
‘The zinc finger platform that Sangamo uses is based off the human C2H2 zinc fingers, of which there are already 350 examples in the human body – so our bodies have already used this as an engineering platform to do gene regulation,’ explains Gregory Davis, Sangamo’s head of research and technology.
‘The thing we’ve been focusing on the most is our zinc finger repressors. They’re small proteins, they have the zinc finger portion, which is usually about six fingers long, and then we attach to it an epigenetic repression domain, typically it’s called a Krab domain,’ says Davis. ‘And the zinc finger will bind the control region of a gene, and this Krab protein will sit there along with the zinc finger at that site, and it will recruit a native complex within the cell that just exists in every human cell to come and methylate in that region and shut the gene down.’
Sangamo is specifically looking at neurological applications for its epigenetic editors. This summer, the company will launch a clinical trial of a treatment for idiopathic small fibre neuropathy (ISFN), a chronic pain syndrome. As the target cells are located in spinal nerves, the editor will be delivered in an adeno-associated virus called AAV9, which has a high affinity for the central nervous system, and will be administered directly into the cerebrospinal fluid.
The drug will target the SCN9A gene, which codes for a particular sodium ion channel (NaV1.7) that is expressed in high levels in pain neurones. Mutations in NaV1.7 are linked to a range of pain disorders, including extreme pain disorders and also congenital insensitivity to pain.
We’re exquisitely specific because we target the DNA – we’re not a small molecule that targets the protein
A major benefit of Sangamo’s approach is that it can target NaV1.7, without impacting other related sodium ion channels. ‘We’re exquisitely specific because we target the DNA – we’re not a small molecule that targets the protein – and we have shown in our animal model and in vitro that we are very specific to NaV1.7 and do not touch the other ones, so that’s really exciting,’ notes Nathalie Dubois-Stringfellow, chief development officer at the company.
The company is also targeting diseases of the brain, but delivering epigenetic editors to brain tissue is especially difficult. Dubois-Stringfellow explains that Sangamo committed significant effort to develop viral capsids that can be injected intravenously, cross the blood–brain barrier and reach a wide range of brain regions.
One year ago, the company revealed preclinical data on an adeno-associated virus capsid variant named Stac-BBB. In non-human primates, the vector delivered cargo to brain tissue 700-times more effectively than AAV9. Dubois-Stringfellow says that this ‘best-in-class’ blood–brain barrier penetrating vector opened the door to a lot of different diseases, and the company is now building a whole pipeline of treatments around it.
First on the list are inherited prion diseases, for which there are currently no treatments. These neurodegenerative illnesses are caused by a misfolding protein that is encoded by a gene called PRNP. So far, Sangamo has shown that it can supress PRNP in animal models, and is planning to apply to begin a human trial in the UK in early 2026.
‘We know from the monkey study that [the drug] goes into the all the regions that are important to target for prion disease,’ says Dubois-Stringfellow. ‘And we also have extraordinary empirical data in a mouse model of prion disease, where we extend the life of mice to a normal life, when they should have died within three months.’
Sangamo’s technology has also been licensed by other biomedical companies. Genentech paid $50 million up front, with a potential $1.9 billion to follow in milestone payments, to license the Stac-BBB system and Sangamo’s zinc-finger repressors to target the tau protein that is linked to Alzheimer’s disease. Astellas and Eli Lilly have also signed similar deals, licensing rights to use Stac-BBB in neurological diseases.
A bright future
‘The chemistry coming out from our genes is written in epigenetics – it’s really the regulatory layer of the DNA,’ says Epic Bio’s Qi. He notes that while companies are establishing the safety of epigenetic editors, they are predominantly focusing on diseases that are well understood. But he believes that in the future, treatments based on precision epigenetic editing could extend to a wide range of other illnesses.
‘In human genetics research, people have found clues or [specific gene] mutations causing about 30 to 40% of diseases,’ he says. ‘But other diseases related to aging, cancer, neurological conditions, Alzheimer’s, you name it – many of them cannot be linked to a clear mutation.’
‘It’s very clear, a lot of these diseases develop while people grow – while people adapt to environments after they are born – and are related to epigenetics. From the medicine side, these are super-high stakes, affecting a lot of people,’ he adds.
‘For now, we’re really focused on the diseases that we understand well, to develop the drugs and prove they are safe and bring them into the human body. But in the future, there are really bright possibilities for how they could be used.’
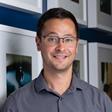
No comments yet