Atomic manipulation has been used to create the most complex molecule ever assembled using this technique. The structure is a molecular wire with a carbon backbone that was manipulated to have as many as eight triple bonds. This new approach to making molecular wires could aid the fabrication of carbon nanoribbons and molecular electronics.
Atomic force microscopy (AFM) allows researchers to mechanically ‘feel’ the contours of single molecules on surfaces and thereby record images of their shapes. Scanning tunnelling microscopy (STM) enables similar imaging feats using the tunnel effect which depends on the variations in very small distances between probe and surface. As the STM requires application of a voltage, it can also serve to add or remove electrons from the molecule being studied, and thereby trigger a reaction. The progress of the reaction can then be monitored in structural terms by AFM which can make use of the same setup.
This is the first time anyone has observed and controlled a skeletal rearrangement at the single-molecule level
Harry Anderson, University of Oxford
This new approach of triggering reactions by applying voltages to specific atoms was pioneered synthetically in 2016 by Leo Gross at IBM Zurich, where both STM and AFM were invented a generation earlier. Together with Diego Peña and colleagues from the University of Santiago de Compostela in Spain, the IBM group focused on Bergmann cyclisation, a reversible interconversion between an anthracene-like triple ring diradical and a version where two of the rings are merged into one that includes two carbon–carbon triple bonds and thus represents a cyclic diyne. The diyne is a promising prodrug that can potentially be used to form the highly potent and reactive diradical in the body.
Using targeted voltage pulses administered through an STM tip, the Zurich researchers could convert a dibromo derivative of the triple ring molecule into the diradical, then into the cyclic diyne, and back to the diradical, each time monitoring the structural changes by AFM.1
Next level
Together with chemists Harry Anderson and Przemyslaw Gawel at the University of Oxford, UK, Gross’ team at IBM has now been able to adapt a similar interconversion between a dibromo alkene, a carbene – like the diradical above, it has two unpaired valence electrons, but this time on the same carbon atom – and an alkyne to take the atomic manipulation approach to new levels of complexity.2
Gawel tells Chemistry World: ‘I heard Leo Gross present his work on the Bergman rearrangement at a conference in Paris, and I realised that the reaction of a 1,1-dibromoalkene to form an alkyne is a similar process – both reactions involve the initial breaking of carbon–bromine bonds, followed by rearrangement. I discussed this with Leo at the conference, and that conversation led to this project.’
This reaction has been known as the Fritsch–Buttenberg–Wiechell rearrangement since 1894, but its mechanism has remained controversial. While some studies suggested that the carbene intermediate has an important role, others pointed to a direct route to the rearrangement.
Single molecule first
In the new study, AFM observation of the successful rearrangement on a sodium chloride surface failed to detect a long-lived carbene intermediate. By contrast, on a copper surface, the reaction does produce the carbene, but it binds to the copper and fails to proceed with the intended rearrangement. Using the STM stimulation on the sodium chloride surface, the researchers first produced a simple alkyne from a dibromoalkene.
‘This is the first time anyone has observed and controlled a skeletal rearrangement at the single-molecule level,’ Anderson says. ‘1,2-shifts such as Wagner–Meerwein rearrangements are important in organic chemistry, so it is interesting to watch one happen. In this case, it is interesting that we see the radical intermediate but don’t see any intermediate carbene.’
The team then went on to synthesise longer chain polyynes with up to eight triple bonds, using dibromo alkenes that already carry alkyne chains on the other carbon. Long conjugated polyynes are of interest both for theoretical considerations regarding the nature of sp hybridised carbon, and for practical use as molecular wires in nanoscale electronics. ‘However, they can be unstable in the bulk, which leads to a motivation for developing methods for fabricating polyynes on surfaces,’ Anderson explains.
Saw-Wai Hla, from Ohio University in the US, who pioneered on-surface reactions by atom manipulation, welcomes the advance as ‘an incredible achievement’. The researchers ‘have constructed individual polyynes on a two-atom thick salt layer one molecule at-a-time’, Hla says. ‘The energetics and images of detailed reaction steps unveiled in this work are not only vital for fundamental understanding of chemistry but also will be useful to find novel paths for on surface synthesis of molecules. I could imagine building new molecular systems that have yet to be discovered using atomic manipulation.’
Johannes Barth at the Technical University of Munich in Germany is also impressed and says that ‘this elegant work conveys a remarkable advance regarding the molecular-level control of chemical conversions and the realisation of all-carbon scaffolds incorporating sp-hybridised segments’.
References
1 B Schuler et al, Nat. Chem., 2016, 8, 220 (DOI: 10.1038/nchem.2438)
2 N Pavliček et al, Nat. Chem., 2018, DOI: 10.1038/s41557-018-0067-y
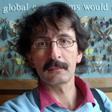
No comments yet