Anthony Green’s research group at the University of Manchester, UK, reengineers enzymes to have catalytic functions beyond those found in nature
Can scientists design and engineer artificial enzymes to make the chemicals that society needs, with the selectivities and efficiencies of natural enzymes? That’s the ultimate question motivating research in Anthony Green’s lab at the University of Manchester, UK.
‘We specialise in designing enzymes that have catalytic functions beyond those found in nature,’ explains Green. His team’s research strategy centres on tailoring enzymes’ structures by using an expanded genetic code. Nature might be restricted to 20 amino acids but that doesn’t mean science should be too.
Genetic code expansion was pioneered by Peter Schultz’s lab, first at the University of California, Berkeley then later at the Scripps Research Institute in the US at the very end of the 20th century. Green’s team uses genetic code expansion to encode new functional groups into proteins. ‘If you want to do new chemistry that nature hasn’t done, a really good way of doing that is to introduce new functional groups,’ explains Green. ‘And because we do that on a genetic level, we can capitalise on the ability to optimise enzymes through either computational design, or through directed evolution, to make systems that are really, really efficient.’
Increasing efficiency is what Green especially enjoys about his work. ‘We take systems from being initially maybe only five to 10-fold more active than the parent small molecule, to hundreds of thousands of fold faster.’
New excited-state chemistry
One functionality that Green and his colleagues have been targeting is triplet energy transfer. Such energy transfer catalysis is a powerful synthetic tool for harnessing light to achieve high selectivity in reactions including isomerisations, cycloadditions and bond cleavage. ‘Ultimately it allows you to construct entirely new carbon frameworks that are very difficult to access other ways,’ explains Green.
‘Nature doesn’t really do triplet energy transfer chemistry. And it certainly doesn’t do stereocontrolled-triplet energy transfer chemistry. It’s really an invention of the organic chemistry community,’ Green notes. ‘We felt that if we could get that kind of chemistry working in proteins, we could end up with a very versatile platform.’
Directed evolution allowed us to find phenotypic traits that are difficult to replicate with small molecule systems
In 2022, Green and his colleagues reported that they had designed and created a photoenzyme for enantioselective [2+2] cycloadditions.1 It was a long project with several variables to work through. First the team had to decide which photosensitiser to use; here they used the amino acid 4-benzoylphenylalanine, which has a benzophenone moiety, although they have since identified photosensitisers with better properties. They had to pick a translation system for embedding the photosensitiser into proteins; here they used an orthogonal aminoacyl-tRNA synthetase/tRNA pair. And they also had to select a protein scaffold; here they used a computationally-designed enzyme for performing Diels–Alder reactions.
To test the system, they shone a light on it. ‘Once we see signs of activity above the small molecule system, or any stereocontrol during the reaction, that’s the place where we’re really happy. We know that even with low levels of activity and stereocontrol, we can develop directed evolution workflows to optimise those catalysts,’ explains Green.
Directed evolution is an iterative process for identifying mutants with new or improved properties; biochemist Frances Arnold received 50% of the 2018 Nobel prize in chemistry in recognition of her work developing the process. Green’s team used an evolutionary strategy involving random and targeted mutagenesis to maximise productive interactions between the protein, substrate and photosensitiser in the hope of improving properties such as activity, selectivity and substrate scope. They ended up with a photoenzyme with five additional mutations that could promote intramolecular and bimolecular cycloadditions, including transformations that small-molecule catalysts have struggled to achieve.
Rarely does Green’s team finish a project without characterising their enzyme to understand why it is so good. ‘In this case we had a really nice crystal structure that showed a lot of the non-covalent interactions that have emerged during evolution. There was really nice π-stacking between the substrate and the sensitiser. And then we saw something we didn’t expect – the system was completely oxygen-tolerant,’ says Green. Normally oxygen is the killer of triplet energy processes. ‘Here directed evolution allowed us to find phenotypic traits that are difficult to replicate with small molecule systems.’
Green’s work unlocking synthetic pathways never seen before in chemistry labs or in nature led to him being named as the chemical science laureate of the 2024 Blavatnik Awards for Young Scientists in the UK, for which he received an unrestricted prize of £100,000. He describes it as a really big honour. ‘As you’re growing up through science you know about the Blavatnik Awards – it’s one of those huge benchmark awards you hope you might apply for at some point.’
However, Green says he doesn’t see the Blavatnik award as a personal award. ‘It’s an award for the group’s research. They drive everything we do. It also gives you the confidence that we’re doing interesting work and moving in a good direction. And it might even attract new scientists to the area.’
References
1 JS Trimble et al, Nature, 2022, 611, 709 (DOI: 10.1038/s41586-022-05335-3)
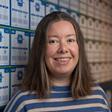
No comments yet