Enzymes are nature’s ultimate catalysts and chemists are now on the verge of making their own versions from scratch
The penultimate holy grail article was penned by Columbia University’s Ronald Breslow. Breslow, who passed away three years ago, was a giant in the field of organic chemistry – a former president of the American Chemical Society, he was well known for his work on the mechanism of action of vitamin B1, aromaticity, remote functionalisation and the origins of homochirality on Earth.
Another major interest of Breslow’s was studying how enzymes carry out reactions so quickly and efficiently, and using this insight to develop new catalysts. In 1970, he coined the term ‘artificial enzyme’, and over several decades his group produced a huge body of work aiming to mimic biocatalytic processes using simpler analogues.
There’s so many opportunities for bringing new chemistry into the biological world
Frances Arnold, California Institute of Technology
‘He was clearly a pioneer in this in this field,’ says University of Basel chemist Thomas Ward. ‘And if you look at the people that have worked with him, or for him and what they’ve gone on to do, he’s certainly been extremely influential.’
Breslow was very interested in cyclodextrins – a family of macrocycles made of multiple glucose subunits. Like enzymes, these incorporate numerous functional groups that can help to coordinate substrates and direct catalysis. Breslow showed that reaction rates could be improved by tailoring the structure and flexibility of the cyclodextrin to better accommodate substrates and transition states.
In one example, Breslow demonstrated that a cyclodextrin could bind two substrates and promote a Diels-Alder cycloaddition. At the time, it was believed that no natural enzyme existed for this reaction, and showed, in Breslow’s words, that ‘biomimetic chemistry [could] go beyond the range of biochemical catalysis’.
Breslow also sought to mimic metal-containing enzymes by incorporating metal-binding groups into his catalysts. Shortly before writing the holy grail paper, Breslow reported an enzyme mimic comprising a lanthanum-binding group connected to two cyclodextrin units. These two groups would stretch substrate molecules across the metal centre allowing it to hydrolyse esters that wouldn’t normally coordinate to metal ions.
In other examples, Breslow attached metalloporphyrins – like those seen in cytochrome P450 enzymes – to cyclodextrin structures, again showing how noncovalent interactions could guide substrates over the metal and facilitate site-selective catalysis. Many researchers have built on these concepts, designing molecular recognition elements into their organometallic catalysts with the aim of imitating enzymes’ behaviours.
Some researchers have taken even more inspiration from nature’s blueprint. Rather than binding a metal into a simplified organic pocket, why not use a readymade biomolecule? The University of Groningen’s Ben Feringa and Gerard Roelfes have pioneered ways to exploit the handedness of DNA helices in new enantioselective catalysts.
Engineering nature
Other groups have returned to proteins. Proteins’ active sites have been carefully crafted over millions of years to carry out chemical transformations with incredible selectivities. Why not just reengineer these for non-natural processes?
Early attempts to modify proteins’ active sites and incorporate new metal-coordinating structures into them were carried out in the labs of Emil Kaiser and George Whitesides in the 1970s. But despite initial promising results, Ward explains that progress was slow. ‘The thing is in the late 70s, it was not trivial to produce recombinantly a protein. And basically this field went dormant for 25 years,’ he says.
Advances in protein expression and genetic engineering brought on a resurgence of interest in protein-based artificial enzymes around the turn of the millennium. In 1999, a group led by Albert Chan at the Hong Kong Polytechnic University, revived Whiteside’s approach to incorporate a rhodium complex into avidin, a glycoprotein found in egg white. The resulting hydrogenation catalyst showed improved, although still modest, enantioselectivities, but more importantly the study signalled a boom in new research into protein-based artificial enzymes. Over the last two decades, researchers around the globe have used many different proteins to create artificial metalloenzymes that catalyse a wide range of reactions. Whiteside’s original paper, which received an average of two citations per year between its original publication in 1978 and Chan’s study in 1999, has been cited almost 400 times since the turn of the century.
One advantage of protein-based artificial enzymes is that they can be used in biological environments. This means that they could potentially be integrated into metabolic processes. ‘In the presence of an enzyme, you cannot add an organometallic catalyst because they’re mutually incompatible,’ says Ward. ‘So both the enzyme inhibits the organometallic catalyst and the organic catalyst inhibits the enzyme. If you compartmentalise the organometallic catalyst in a protein, then these become compatible.’
In 2012, Ward’s group showed how an artificial enzyme comprising an iridium complex embedded in a streptavidin host protein could work in tandem with natural enzymes in a multi-step cascade reaction. Building on this, they’ve since used an artificial metalloenzyme to regulate a gene circuit inside a mammalian cell.
Controlling evolution
Another significant benefit to working with proteins is that they can be optimised using directed evolution techniques – something biochemist Frances Arnold at the California Institute of Technology won the 2018 chemistry Nobel prize for.
‘There’s so many opportunities for bringing new chemistry into the biological world. Nature has her choice of reactivities – but that doesn’t mean she’s limited to those,’ says Arnold. ‘So I think the very exciting opportunities will come from chemists who look at enzymes with a whole new set of eyes, not for what they do now, but for what they could do – especially if you train them with evolution.’
Arnold’s lab has shown how directing rounds of mutations can instil new functionality in enzymes. The group has created enzymes that can convert broad ranges of substrates and perform numerous non-natural reactions, from cyclopropanation to carbon–silicon bond formation.
In one example, Arnold’s group showed how mutations introduced to a monooxygenase enzyme could enable it to efficiently catalyse nitrene transfer reactions. This non-native chemistry was first predicted by Breslow during his early investigations of haem proteins.
‘It all comes back to Ron Breslow. He proposed in 1985 that a cytochrome P450 can do nitrene transfer … and in fact, he showed that it could do one or two turnovers,’ says Arnold. ‘Twenty-five years later, we had the tools to take that little tiny bit of reactivity and turn it into a real enzyme, but it was his inspiration that drove that discovery.’
For Arnold, this illustrates the new possibilities that can arise when chemists look to build upon nature’s designs. ‘A biochemist is looking at the protein for what it does in a biochemical system, [whereas] the chemist doesn’t care, right? The chemist says, “Oh, here’s a beautiful active site that nature has made – what could it do?”,’ she explains. ‘And that’s what biomimetic chemistry was trying to do – not just mimic nature, but also think of new things. So that gave us a tremendous amount of inspiration.’
When chemistry meets biology
Now, researchers like Ward are making use of directed evolution while also augmenting enzymes with new metal-containing catalytic centres. ‘Gerald Joyce once claimed very insightfully that “biological systems have a molecular memory”,’ says Ward. ‘The point is the memory allows you to optimise the performance because you have this link between the phenotype and the genotype. What occurred to me much after I’d started in this topic, was that what we’re doing is adding a genetic memory to organometallic chemistry.’
Recently, John Hartwig’s group at the University of California, Berkley, developed efficient ways to replace the iron-containing porphyrin in haem proteins with cofactors containing noble metals. By doing so, they expanded the types of chemistry these enzymes can carry out. Their iridium-containing artificial enzymes can catalyse carbon–carbon bond forming reactions that the natural iron-containing haem proteins won’t promote. By combining this strategy with directed evolution they’ve been able to enhance the enzyme’s activity to match the rates and selectivities seen in native enzymes.
Another of the groups leading the way is the ETH Zurich lab of Donald Hilvert, who previously worked with both Breslow and Kaiser. Hilvert’s team has even created highly active metalloenzymes from scratch. In 2018, they generated a small protein from a computationally designed zinc-binding peptide, and then used directed evolution to drive its activity for ester hydrolysis reactions. The resulting enzyme carried out this function with rates comparable to those seen in natural enzymes. For Ward, this demonstrates the power of blending chemistry and biology.
‘If you take a hydrolase, whose rate approaches 108 turnovers per second, and you create an artificial metalloenzyme from scratch that has such rates, I would argue you have shown that it is indeed possible to endow organometallic chemistry with a genetic memory,’ say Ward. ‘I think this is really quite spectacular.’
Geo caption: This map shows the locations of researchers who have authored the most cited papers on artificial enzymes over the last 25 years. The chart shows a broad geographic spread, with high concentrations of researchers based in the US and central Europe. The emergence of important papers from China in later years may also reflect the growing interest in ‘nanozymes’ – nanomaterials with enzyme-like properties.
Cumulative cites caption: The top 20 papers on artificial enzymes since 1995, in terms of citations. Top place goes to a 2013 review article on nanozymes that has been cited 1336 times. Ronald Breslow appears twice with review papers – including the holy grail paper – on cyclodextrin-based enzyme mimics. In ninth place (D Rothlisberger et al) is a paper from Dan Tawfik and David Baker’s labs on computational enzyme design.
Citation network caption: This chart shows how researchers working on artificial enzymes are connected by analysing who they tend to cite in their papers. The main hub is dominated by researchers who work on protein engineering and biocatalysis, with Frances Arnold and Manfred Reetz appearing as the largest nodes due to the high number of citations their work attracts. Ward, Roelfes and Feringa can be found in the smaller cluster to the right of the main group. The group to the far right of the chart includes many researchers with interests in catalytic nanomaterials or ‘nanozymes’, with Jinsong Ren and Xiaogang Qu appearing as two of the largest nodes. Breslow, whose work on organic enzyme mimics is somewhat different from these other areas can be found near the top right corner of the chart.
Co-author network caption: In this chart, researchers are connected to other researchers that they have co-authored papers with. It allows us to visualise groups who have worked and collaborated closely with each other. Frances Arnold and Manfred Reetz who are both well known for their pionnering work on enzyme evolution are the who largest nodes in the centre of the chart. Just below the centre of the chart we can see the Weizmann Institute’s Dan Tawfik and ETH Zurich’s Donald Hilvert who are connected via University of Washington research David Baker, with whom both have published papers on computational protein design.
Additional information
For an explanation of how these data visualisations were built, read Behind the data
Further reading
1 P Zhang et al, Exploration of advanced porous organic polymers as a platform for biomimetic catalysis and molecular recognition, Chem. Commun., 2020, 56, 10631 (DOI: 10.1039/d0cc04351f)
2 D Z Zee and T D Harris, Enhancing catalytic alkane hydroxylation by tuning the outer coordination sphere in a heme-containing metal–organic framework,Chem. Sci., 2020, 11, 5447 (DOI: /10.1039/d0sc01796e)
3 W Wu et al, Atomic engineering of single-atom nanozymes for enzyme-like catalysis, Chem. Sci., 2020, DOI: 10.1039/d0sc03522j
4 J J Garrido-González et al, An enzyme model which mimics chymotrypsin and N-terminal hydrolases, ACS Catal., 2020, DOI: 10.1021/acscatal.0c02121
5 M D Nothling et al, A multifunctional surfactant catalyst inspired by hydrolases, Sci. Adv., 2020, 6, eaaz0404 (DOI: 10.1126/sciadv.aaz0404)
6 N Ch Jana et al, Facile biomimetic catalytic activity through hydrogen atom abstraction by the secondary coordination sphere in manganese(iii) complexes, Dalton Trans., 2020, DOI: 10.1039/d0dt02431g
7 D Wang et al, Origins of the peroxidase mimicking activities of graphene oxide from first principles, J. Mater. Chem. B, 2020, DOI: 10.1039/d0tb01765e
8 W J Jeong, J Yu and W J Song, Proteins as diverse, efficient, and evolvable scaffolds for artificial metalloenzymes, Chem. Commun., 2020, 56, 9586 (DOI: 10.1039/d0cc03137b)
9 F Wang et al, A mesoporous encapsulated nanozyme for decontaminating two kinds of wastewater and avoiding secondary pollution, Nanoscale, 2020, 12, 14465 (DOI: 10.1039/d0nr03217d)
10 Z Zhou and G Roelfes, Synergistic catalysis in an artificial enzyme by simultaneous action of two abiological catalytic sites, Nat. Cat., 2020, 3, 289 (DOI: 10.1038/s41929-019-0420-6)
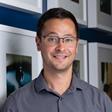
Searching for the holy grails of chemistry
- 1
- 2
- 3
- 4
- 5
- 6
- 7
- 8
Currently reading
Designer enzymes offer a way to learn from and even surpass nature’s genius
- 9
No comments yet