A dream since electricity was first harnessed humanity is tantalisingly close to achieving this goal
Room temperature superconductors were put forward by solid state chemist Arthur Sleight as another ‘holy grail of chemistry’ in 1995. Over the last quarter of a century, this field has seen huge advances and the dream of room temperature superconductivity seems tantalisingly within reach.
It just blows your mind that you can get a material that conducts electricity or electrons without losing energy
José Flores-Livas, Sapienza University of Rome
Superconductors are materials that exhibit no electrical resistance. Their unusual properties have made them key components in quantum computers, particle accelerators and NMR magnets. But most materials only display superconductivity at very low temperatures, requiring expensive and energy-consuming cooling systems. Many scientists believe that the ability to exploit superconductivity at higher temperatures would allow extraordinary new applications, perhaps transforming the way we distribute electricity.
‘It just blows your mind that you can get a material that conducts electricity or electrons without losing energy. For me, it’s just incredible,’ says Jose Flores-Livas, who develops computational tools to explore superconducting materials at Sapienza University of Rome. He points out that while the room temperature target of 300K is somewhat arbitrary, it does have significance. ‘There is no theory that says you cannot have [superconductivity] at 100K, 250K or even 600K,’ says Flores-Livas. ‘But it’s more like a landmark that we human beings have said “okay if those materials can be compatible with us in the thermodynamic conditions that we live in, it’s kind of special” – because then these materials will allow many technological revolutions.’
A chemistry problem
At the time of Sleight’s paper, a family of copper-based materials known as the cuprates were making waves in the superconductivity community. The cuprates appear to break all of the rules on superconductivity, and were pushing critical temperatures (Tc) – the point where superconductivity manifests– to temperatures never before seen. The first cuprate was lanthanum barium copper oxide, which was discovered in 1986 with a record-breaking Tc of 35K. By 1995, the record Tc was held by a Hg–Ba–Ca–Cu–O system, which displayed superconductivity at 135K. Unlike conventional superconductors, superconductivity in the cuprates can’t be explained by Bardeen–Cooper–Schrieffer (BCS) theory – the governing rules of superconductivity that won the 1972 Nobel prize in physics.
‘I would say that most of us interested in superconductivity are still waiting for an explanation from theorists of what the mechanism is for superconductivity in these cuprates,’ says Sleight. He explains that, while the basis of superconductivity in conventional superconductors is well accepted, the mechanism in the cuprates is highly controversial. ‘I remember the first year after the discovery of high Tc in the cuprates the theorists were all telling me: “Well,” they said, “all the best theorists in the world had dropped what they’re doing, and they’re all working on this one problem and they’re going to have an answer very, very soon – within a year!” And of course it’s now more than 30 years later, and there’s no consensus at all what is making these materials superconducting.’
The cuprates are remarkable in that they achieve high-temperature superconductivity at atmospheric pressure – a combination of properties that led to practical uses, for example in superconducting cuprate wires currently used at Cern’s Large Hadron Collider. As superconducting complex mixtures of multiple elements, the cuprates also helped to open up high temperature superconductivity as a chemistry challenge, rather just a question for theoretical physicists. ‘Most people think of this as a problem in physics, because it’s a physics problem fundamentally discovered in simple systems – in metals, simple alloys and so on, going back to the very early years,’ says Russell Hemley, who researches high pressure behaviour of materials at the University of Illinois, US. ‘But with the discovery of the cuprates … the chemical complexity of those systems, I think, reminded people of the problem as being one more broadly in physical sciences – where chemistry plays a very important role.’
This led to discoveries of other multi-element superconducting systems – in particular iron-based materials in the early 2000s that achieved critical temperatures near those of the cuprates. But the next big jump wouldn’t come until 2015 and it wouldn’t come from a cuprate, or any of the elements known for their conductivity.
The first element
Running parallel with the search for room temperature superconductors has been the quest to create metallic hydrogen. A metallic phase of hydrogen that was first predicted in the early 20th century, which would only exist under extremely high pressures – equivalent to those experienced at the centre of a planet. In 1968, metallic hydrogen was calculated to be a very high Tc superconductor, and this led researchers to begin exploring other hydrogen-rich compounds.
But high pressure experiments are not easy, or cheap. High purity materials must be compressed between precisely cut, single-crystal diamond anvil cells. Measuring what takes place inside the cell is not simple either, although developments with miniature electrodes and modern synchrotron light sources have dramatically increased our ability to determine what is happening.
In the 2000s it became possible to reach pressures of 200GPa – around 200 million atmospheres – in diamond anvil cells. Today, pressures exceeding 1000GPa have been reported using specially synthesised nanodiamonds.
Given the cost and complexity, it’s simply not possible to run high-throughput screenings of every combination of materials, and so modern computational methods have become key to identifying which experiments to try. The accuracy with which computational techniques can now predict superconducting phases of materials has sparked a new era in the race to create high Tc superconductors.
In 2014, a group led by Yanming Ma at Jilin University in China predicted that, under extreme pressures, hydrogen sulfide (H2 S) would become a very high temperature superconductor. In 2015, experiments on hydrogen sulfide carried out by researchers at the Max Planck Institute for Chemistry in Mainz, Germany, showed that at pressures around 150GPa, hydrogen sulfide would transition into a superconducting form (H3 S), with a Tc of 203K. This culminated in Hemley’s group observing a Tc of 260K in LaH10 at 188GPa – the current record for any superconductor.
Within reach
Now that binary hydrides have been extensively searched, researchers are beginning to look at more complex materials containing more elements. ‘It’s very exciting,’ says Hemley. ‘And again, it’s a problem in chemistry – it’s a problem in combinatorial chemistry because we have all these different combinations.’ Hemley believes that the vast new chemical space opened up by the ternary hydrides presents an opportunity for modern computational techniques to come to the fore. ‘Maybe it’s an opportunity for deep learning and machine learning approaches here, because there’s a lot of data we’ll need to sort through to look for trends to optimise properties,’ he says.
There are predictions now that critical temperatures of ternary hydrides could go way beyond room temperature into the realms of ‘hot superconductivity’. Last year Yanming Ma’s group at Jilin University in China calculated that at 250GPa, lithium magnesium hydride would form a superconducting phase with a Tc of 473K. ‘That’s of course just astonishing, but it is done at the same level of theory that got the right answer for lanthanum hydride, so we need to take it seriously,’ says Hemley. ‘It’s a difficult material to make, but it’s probably just representative of a whole class of ternaries out there.’
Hemly also believes that it’s possible that the ternaries may offer a route to produce high temperature superconducting hydrides that remain stable under lower pressures.
So will we break the room temperature barrier soon? Yes, says Flores-Livas, as long as we don’t care about how much pressure is required. ‘I’m pretty sure that someone will measure a very high Tc – about 280–290K – in the next two or three years.’
Of course, high pressure is a barrier to practical uses – so is it likely that we’ll find a material that is superconducting at high temperatures, but stable at low pressures? Looking back at the cuprates and iron superconductors, Flores-Livas is hopeful and points out that every 10 years or so new discoveries have pushed the limits of critical temperatures in ambient systems.
Sleight, meanwhile, says that a room temperature superconductor could already exist. ‘It’s possible that we have room temperature superconductors sitting around somewhere that we don’t know are room temperature superconductors,’ he says. ‘That’s because if you try to measure their properties the way you would normally measure their properties, say, if you’re measuring magnetic properties under some fields, your magnetic fields may be too high, and it may have suppressed the superconductivity at room temperature.’
Co-author network caption: In this chart, each node is a researcher who has authored one of the most cited papers on high-temperature superconductors that have been published within the last 25 years. Researchers with larger nodes have contributed to more of these papers, while the lines connect networks of researchers who have authored papers with each other. Strikingly, this chart shows a broad distribution of names, most of whom are associated with work on cuprate superconductors. This reflects the surge in interest in cuprates through the 1990s and the fact that these materials are now used for various applications. In the coming years, we might expect to see researchers linked to recent work on the superhydrides gain more prominence as their papers accumulate citations.
Citation network caption: The network of citations shows researchers who have been cited in the top papers on high-temperature superconductors from the last 25 years. Like the co-author network, this chart is also dominated by researchers associated with the cuprate superconductors. The largest node belongs to Shin-Ichi Uchida, a Japanese condensed-matter physicist who has been a major player in research into superconductivity in the cuprates since it was first discovered in the mide 1980s. Another prominent researcher is 1977 physics Nobel prize-winner Philip Anderson, whose resonating valence bond theory attempted to describe high temperature superconductivity in the cuprates.
Map caption: This map shows the broad global interest in high-temperature superconductivity. The timeline appears to reflect the peak interest in copper and then iron-based superconductors in the late 1990s and early 2000s. Key papers on the superhydrides are too recent to have broken in to the top 500 papers for this broad area of research.
Top cited papers caption: This chart shows the 20 most highly cited papers on high temperature superconductivity that have been published since 1995. Top place, with 2450 citations, goes to a review focusing on experimental analyses of cuprate superconductors. The fourth most cited paper (1490 cites) is a 2008 report on the discovery of an iron superconductor with a transition temperature of 43K – a record at the time for non-cuprate materials. Racing into eleventh position, despite only being published five years ago, Aleksander Drozdov and co-workers’ paper heralded the dawn of the hydrides, with the first experimental observation of superconductivity at 203K in sulfur hydride.
Additional information
For an explanation of how these data visualisations were built, read Behind the data
Further reading
1 M Kostrzewa, From LaH10 to room–temperature superconductors, Sci. Rep., 2020, 10, 1592 (DOI: 10.1038/s41598-020-58065-9)
2 T Yoon, J Y Koo and H C Choi, High yield organic superconductors via solution-phase alkali metal doping at room temperature, Nano Letters, 2020, 20, 612 (DOI: 10.1021/acs.nanolett.9b04377)
3 I A Kruglov et al, Superconductivity of LaH10 and LaH16 polyhydrides, Phys. Rev. B, 2020, 101, 024508 (DOI: 10.1103/PhysRevB.101.024508)
4 1 I Errea et al, Quantum crystal structure in the 250-kelvin superconducting lanthanum hydride, Nature, 2020, 578, 66 (DOI: 10.1038/s41586-020-1955-z)
5 S Di Cataldo, W von der Linden and L Boeri, Phase diagram and superconductivity of calcium borohyrides at extreme pressures, Phys. Rev. B, 2020, 102, 014516 (DOI: 10.1103/PhysRevB.102.014516)
6 A Sanna, C Pellegrini and E K U Gross, Combining Eliashberg theory with density functional theory for the accurate prediction of superconducting transition temperatures and gap functions, Phys. Rev. Lett., 2020, 125, 057001 (DOI: 10.1103/PhysRevLett.125.057001)
7 A Amon et al, Y4 Be33 Pt16 – a non-centrosymmetric cage superconductor with multi-centre bonding in the framework, Dalton Trans., 2020, 49, 9362 (DOI: 10.1039/d0dt01374a)
8 A Yamashita et al, An efficient way of increasing the total entropy of mixing in high-entropy-alloy compounds: a case of NaCl-type (Ag,In,Pb,Bi)Te1−x Sex (x = 0.0, 0.25, 0.5) superconductors, Dalton Trans., 2020, 49, 9118 (DOI: 10.1039/d0dt01880e)
9 X Yang et al, Observation of short-range Yu-Shiba-Rusinov states with threefold symmetry in layered superconductor 2H-NbSe2, Nanoscale, 2020, 12, 8174 (DOI: 10.1039/d0nr01383h)
10 C Liu et al, Superconductivity in compression-shear deformed diamond, Phys. Rev. Lett., 2020, 124, 147001 (DOI: 10.1103/PhysRevLett.124.147001)
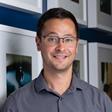
Searching for the holy grails of chemistry
- 1
- 2
- 3
Currently reading
Room temperature superconductivity is now within touching distance – but it won’t change the world yet
- 4
- 5
- 6
- 7
- 8
- 9
No comments yet